Introduction: Complete Guide for Tech Beginners
Every starting is heard but if you have the motivation and strong determination you will be successful. Most of the students especially of the students of engineering want to build and make something. Practical experience of making something makes our learning perfect and help us to understand the real facts. Though it is very much important but most of the students do not get proper guidelines and they do not not from where he should start. I believe this tutorial will help tech beginners and hobbyists to start any project.
To buy any kind of components you may visit:
Step 1: Voltage, Current, Resistance
These are very fundamental things of electronics and I know you are already familiar to these. Lets just recall.Every matter is made of atoms and an atom has three types of particles. Electron is one of the particle among them and is negatively charged. Charge is a property of electron and proton. Protons are positively charged. The charge of an electron is called unit charge. In a conductive material (silver, copper, gold, aluminium etc) there are lots of free electrons which move randomly. Voltage is a force or presser which forced electrons to flow or move in a particular direction. When voltage is applied into a conductor electrons start to move in a fixed direction and flow of electrons in a particular direction is called current. When electrons move in a conductor they face some friction. This friction is called resistance. Resistance oppose the free movement of electrons. So, we can say resistance reduce the current.
Voltage: Voltage is the presser which force the electrons to flow in a particular direction in a conductor. The unit of voltage is Volt and denoted by V. Battery is a good source of voltage. 3V, 3.3V, 3.7V and 5V are most common in electronic circuits and devices.
Current: Current is the flow of electrons in a particular direction. More formally, current is the rate of change of electron in a particular direction. Unit of current is Ampere and denoted by I. In electronic circuits current is in milliampere range (1 Ampere = 1000 milliampere). For example, typical current for an LED is 20mA.
Voltage is the cause and current is the result.
Resistance: Resistance is the hindrance to the flow of charge or electron. The unit of resistance is The SI unit of electrical resistance is the ohm (Ω).
There is an important relationship among these three quantity voltage, current and resistance:
V = IR or I = V / R or R = V / I
This relation is called Ohm's Law. Voltmeter, ammeter and ohm meter is used to measure voltage, current and resistance respectively.
Step 2: Battery
An electric battery is a source of voltage or more formally source of electrical energy. Battery provides electrical energy by internal chemical reaction. Battery is a two terminal device. One is call positive terminal (+V) and another is negative terminal (-V) or ground. Generally batteries are two types.
- Primary
- Secondary
Primary batteries are used once and discarded. Secondary batteries can be discharged and recharged multiple times. Batteries come in many shapes and sizes, from miniature cells used to power hearing aids and wristwatches to battery banks the size of rooms that provide standby power for telephone exchanges and computer data centers. According to battery chemistry battery can be many types. Several common types of battery used in robotics and tech project are discussed below.
1.5 V battery
Different size 1.5 V battery are available. Most common sizes are AA and AAA. Capacity range is 500 to 3000 mAh.
3 V Lithium coin cell
Coin-shaped cells are thin compared to their diameter.All these lithium cells are rated nominally 3 volts (on-load), with open circuit voltage about 3.6 volts. Capacity may very from 30 to 500 mAh. Extensively used in wearable device for their tiny size.
Alkaline
These batteries are not re-chargeable and could be used in small robots.
Nickel-Metal Hydride (NIMH)
These batteries, has a high energy density, and can be charged quickly. Another important feature is the price. NIMH batteries are cheap for their size and capacity. This type of battery is frequently used in robotic applications.
3.7 V Li-ion and Li-polymer
Lithium ion and lithium polymer batteries are rechargeable. They have high discharging capacity, high energy density, high capacity and small size. Li-polymer battery are used extensively in robotics and RC project. Specific energy of Li-poly battery is 100–265 W·h/kg.
9 V battery
Nine-volt battery in its most common form was introduced for the early transistor radios. It has a rectangular prism shape with rounded edges and a polarized snap connector at the top. They all have a rectangular shape; the dimensions are height 48.5 mm, length 26.5 mm, width 17.5 mm (or 1.9"x1.0"x0.68"). Both terminals are at one end and their centers are 1/2 inch (12.7 mm) apart. Capacity is around 600 mAh.
Lead Acid
Lead Acid batteries are the workhorse batteries of industry. They are incredibly cheap, rechargeable, and easily available. Lead acid batteries are used in machinery, UPS's (uninterruptable power supply), robotics, and other systems where a lot of power is needed and weight is not as important. Lead acid batteries come in 2V cells, that means you can have a battery with an even number of volts. The most common voltages are 2V, 6V, 12V and 24V.
Series parallel connection of battery
Battery can be connected to series or parallel. When connected in series the voltage level increased and when connected on parallel the current capacity increased.
Two important things of battery:
Battery Capacity: Battery capacity is a measure (typically in Amp-hr) of the charge stored by the battery, and is determined by the mass of active material contained in the battery. The battery capacity represents the maximum amount of energy that can be extracted from the battery under certain specified conditions. However, the actual energy storage capabilities of the battery can vary significantly from the "nominal" rated capacity, as the battery capacity depends strongly on the age and past history of the battery, the charging or discharging regimes of the battery and the temperature.
Battery capacity is measured in either watt-hours (Wh), kilowatt-hours (kWh), ampere-hours (Ahr) or milliampere-hour (mAh). A Watt-hour is the voltage (V) that the battery provides multiplied by how much current (Amps) the battery can provide for some amount of time (generally in hours). Voltage * Amps * hours = Wh. Since voltage is pretty much fixed for a battery type due to its internal chemistry (alkaline, lithium, lead acid, etc), often only the Amps*hour measurement is printed on the side, expressed in Ah or mAh (1000mAh = 1Ah). To get Wh, multiply the Ah by the nominal voltage. For example, lets say we have a 3V nominal battery with 1Amp-hour capacity, therefore it has 3 Wh of capacity. The battery capacity will be better with lower drain currents. To determine the battery life, divide the capacity by the actual load current to get the hours of life. A circuit that draws 10 ma powered by a 9 volt rectangular battery will operate about 50 hours: 500 mAh /10 mA = 50 hours.
In many types of batteries, the full energy stored in the battery cannot be withdrawn (in other words, the battery cannot be fully discharged) without causing serious, and often irreparable damage to the battery. The Depth of Discharge (DOD) of a battery determines the fraction of power that can be withdrawn from the battery. For example, if the DOD of a battery is given by the manufacturer as 25%, then only 25% of the battery capacity can be used by the load.
The charging/discharging rates affect the rated battery capacity. If the battery is being discharged very quickly (i.e., the discharge current is high), then the amount of energy that can be extracted from the battery is reduced and the battery capacity is lower. Alternately, is the battery is discharged at a very slow rate using a low current, more energy can be extracted from the battery and the battery capacity is higher. For example, a coin cell that is rated for 1 Ah can't actually provide 1 Amp of current for an hour, in fact it cant even provide 0.1 Amp without overextending itself. Its like saying a human has the capability to travel up to 30 miles: of course running 30 miles is a lot different than walking! Likewise, a 1Ah coin cell has no problem providing a 1mA for 1000 hours but if you try to draw 100mA from it, it'll last a lot less than 10 hours.
The temperature of a battery will also affect the energy that can be extracted from it. At higher temperatures, the battery capacity is typically higher than at lower temperatures. However, intentionally elevating battery temperature is not an effective method to increase battery capacity as this also decreases battery lifetime.
C-rate: The charge and discharge current of a battery is measured in C-rate. Most portable batteries, with the exception of the lead acid, are rated at 1C. A discharge of 1C draws a current equal to the rated capacity. For example, a battery rated at 1000mAh provides 1000mA for one hour if discharged at 1C rate. The same battery discharged at 0.5C provides 500mA for two hours. At 2C, the same battery delivers 2000mA for 30 minutes. 1C is often referred to as a one-hour discharge; a 0.5C would be a two-hour, and a 0.1C a 10 hour discharge.
The capacity of a battery is commonly measured with a battery analyzer. If the analyzer’s capacity readout is displayed in percentage of the nominal rating, 100 percent is shown if 1000mA can be drawn for one hour from a battery that is rated at 1000mAh. If the battery only lasts for 30 minutes before cut-off, 50 percent is indicated. A new battery sometimes provides more than 100 percent capacity. In such a case, the battery is conservatively rated and can endure a longer discharge time than specified by the manufacturer.
Charge rate is often denoted as C or C-rate and signifies a charge or discharge rate equal to the capacity of a battery in one hour.
A battery charger may be specified in terms of the battery capacity or C rate; a charger rated C/10 would return the battery capacity in 10 hours, a charger rated at 4C would charge the battery in 15 minutes. Very rapid charging rates, 1 hour or less, generally require the charger to carefully monitor battery parameters such as terminal voltage and temperature to prevent overcharging and damage to the cells.
How Batteries Are Measured
Size
This is pretty straight forward, how big are the batteries? Lead acid batteries don't get much smaller than C-cell batteries. Coin cells don't get much larger than a quarter. There are also standard sizes, such as AA and 9V which may be desirable.
Weight and power density
This is a performance issue: higher quality (and more expensive) batteries will have a higher power density. If weight is an important part of your project, you will want to go with a lighter, high-density battery. Often this is expressed in Watts-hours per Kilogram.
Price
Price is pretty much proportional to power-density (you pay more for higher density) and proportional to power capacity (you pay more for more capacity). The more power you want in a smaller, lighter package the more you will have to pay.
Voltage
The voltage of a battery cell is determined by the chemistry used inside. For example, all Alkaline cells are 1.5V, all lead-acid's are 2V, and lithiums are 3V. Batteries can be made of multiple cells, so for example, you'll rarely see a 2V lead-acid battery. Usually they are connected together inside to make a 6V, 12V or 24V battery. Likewise, most electronics use multiple alkalines to generate the voltage they need to run. Don't forget that voltage is a 'nominal' measurement, a "1.5V" AA battery actually starts out at 1.6V and then quickly drops down to 1.5 and then slowly drifts down to 1.0V at which point the battery is considered 'dead'.
Re-usability
Some batteries are rechargable, usually they can be recharged 100's of times.
Which battery is perfect for your project?
You see there are many types of battery and many types of battery chemistries, so it's not easy to decide on which solution is best for your project. If your project is very power-hungry such as large sound systems, and motorized projects you can select lead acid battery. If you want to build wearable project and required small power you can select lithium coin cell. For any portable light weight project which need moderate power you can select lithium-ion battery. You can also choose cheaper Nickel-Metal Hydride (NIMH), a little heavier in weight compare to li-ion. If you like to make power-hungry RC quad-copter or like that probably Lithium Ion Polymer(LiPo) would the best option for you because these batteries have small dimensions, are lightweight compared with other types of batteries, can be recharged very quickly, and has a high current output.
If your battery need to be user replaceable then you should select universal AA, AAA or 9V battery. If you require 5V battery then you can use 3 AAA or AA alkaline cells (4.5V) or 4 NiMH cells (4.8V). Be sure your device will run at these slightly lower voltages (most probably it does).
Want your rechargable batteries to last a long time?
Use a high-quality charger that has sensors to maintain proper charging and trickle charging. A cheap charger will kill off your cells.
Excellent batteries are available at: http://www.hobbyking.com
Step 3: Resistor
Resistor is an electric component which resists the flow of electron. Resistor is a very basic and most common circuit element. We use resistor to control the current flow in an electric circuit. Controlling current is very important and electronic engineering is nothing but an art of controlling current. You can not find any electric device or circuit without resistor.
Resistors are passive components, meaning they only consume power (and can’t generate it). Resistors are usually added to circuits where they complement active components like op-amps, microcontrollers, and other integrated circuits. Commonly resistors are used to limit current, divide voltages, and pull-up I/O lines.
The electrical resistance of a resistor is measured in ohms. larger or smaller values of ohms can be matched with a prefix like kilo-, mega-, or giga-, to make large values easier to read. It’s very common to see resistors in the kilohm (kΩ) and megaohm (MΩ) range (much less common to see miliohm (mΩ) resistors). For example, a 4,700Ω resistor is equivalent to a 4.7kΩ resistor, and a 5,600,000Ω resistor can be written as 5,600kΩ or (more commonly as) 5.6MΩ.
The Different Types of Resistor
There are many thousands of different Types of Resistor and are produced in a variety of forms because their particular characteristics and accuracy suit certain areas of application, such as High Stability, High Voltage, High Current etc, or are used as general purpose resistors where their characteristics are less of a problem.
Some of the common characteristics associated with the humble resistor are; Temperature Coefficient, Voltage Coefficient, Noise, Frequency Response, Power as well as a resistors Temperature Rating, Physical Size and Reliability.
Based on the conductive properties of a resistor resistors can be classified as:
Linear Resistor: A linear resistor is the type of resistor whose resistance remains constant with increase in the potential difference or voltage applied to it. Or the Resistance or Current passed through the resistor does not changes as the applied voltage ( P.D ) changes. The V-I characteristics of such resistor is a straight line.
Non Linear Resistor: Non-Linear Resistor are those types of resistors in which the Current passed through it is not exactly directly proportional to the Potential Difference applied to it. These types of resistors have non-liner V-I characteristics and does not strictly follows ohm’s Law.
There are several types of nonlinear resistors, but the most commonly used include : NTC resistors (Negative Temperature Co-efficient) - their resistance lowers with temperature rise. PTC resistors (Positive Temperature Co-efficient) - their resistance increases with the temperature rise. LDR resistors (Light Dependent Resistors) - their resistance lowers with the increase in light. VDR resistors (Voltage dependent Resistors) - their resistance critically lowers as the voltage exceeds a certain value.
Non linear resistors are used in different projects. LDR is used as a sensor in various robotics an hobbyist project.
Based on Resistor’s Value
Fixed Value Resistor:
Fixed value resistors are those types of resistors whose value is fixed already while manufacturing and cannot be changed during it’s usage.
Variable Resistor or Potentiometer :
Variable Resistors or Potentiometers are those types of resistors whose Value can be changed during it’s usage. These types of resistor usually contains a shaft which can be rotated or moved by hand or a screw driver to change it’s value in between a fixed range for eg. 0 Kilo Ohms to 100 Kilo Ohms.
Potentiometers are used for volume and speed control in different projects and devices.
Package Resistor :
These types of resistor is a resistor which contains a package which contains two or more resistors inside it. It have many terminals and the resistor’s resistance can be chosen by using any two terminals among the available terminals or can also be used as an resistor array for various purposes.
Based On Composition :
Carbon Composition:
These types of resistors are made by a composition of Carbon Particles which are hold together by a binding resign. The proportion of carbon particles and resign used determines the value of the resistor. At both ends of the composition a Metal Cap with a small rod of tin is attached to solder it or use it in circuits , then the whole package is enclosed in a plastic case to prevent moisture and reaction with air.
These types of resistors normally produces noise in circuit due to electron passing through one carbon particle to another , thus these types or resistors are not used in critical circuits although they are cheap.
Carbon Deposition:
The resistor which is made by depositing a thin layer of carbon around a ceramic rod is called Carbon Deposition resistor. They are made by heating a ceramic rod inside a flask of methane and depositing the carbon around it by using Glass Cracking process. The value of resistor is determined by the amount of carbon deposited around the ceramic rod.
Metal Film:
Metal film resistors are made by depositing vaporized metal in vacuum on a ceramic core rod. these types of resistors are very reliable , have high tolerance and also have high temperature coefficient. These types of resistors are costlier compared to other but are used in critical systems.
Wire Wound:
Wire wound resistor are made by winding a metal wire around a ceramic core. The metal wire is an alloy of various metals based on the characteristics and resistance of the resistor required. These types of resistor have high stability and can also withstand high powers but are usually bulkier compared to other types of resistors.
Ceramic Metal:
These types of resistor are made by firing certain metals blended with ceramics on a ceramic substrate. The proportion of the mixture in the blended ceramic and metal determines the value of the resistor. These type of resistors are very stable and also have accurate resistance. These types of resistors are mostly used as Surface Mount type resistor for using in SMD PCB.
Based on Resistor’s Function:
Precision Resistors:
Precision Resistors are resistors that have very low tolerance values, so they are very precise (closely near
their nominal value).
All resistors come with a tolerance value, which is given as a percentage. The tolerance value tells us how close to the nominal value a resistance can vary. For example, a 500Ω resistor that has a tolerance value of 10%, may have a resistance that is somewhere between 10% above 500Ω (550Ω) or 10% below 500Ω (450Ω). If the same resistor has a tolerance of 1%, its resistance will only vary by 1%. Therefore, a 500Ω resistor can then vary between 495Ω and 505Ω. This is tolerance as an example.
A precision resistor is a resistor that has a tolerance level as low as 0.005%. This means a precision resistor will only vary 0.005% from its nominal value.
Precision resistors have very low tolerance percentage values, making them ultraprecise to their nominal values. They will vary very little from their nominal values, so they are used in applications where high-precision is necessary in terms of resistance values.
Fusible Resistor:
A Fusible Resistor is a wire-wound resistor that is designed to burn open easily when the power rating of the resistor is exceeded. In this way, a fusible resistor serves dual functions. When the power isn't exceeded, it serves as a resistor limiting current. When the power rating is exceeded, it functions as a fuse, burning up, and becoming an open in the circuit to protect components in the circuit from excess current.
Cement Resistors:
Cement resistors are power resistors that are heat and flame resistant. Cement resistors are made to handle a large amount of power flowing through it with it being undamaged by heat or flames. If you are designing a circuit where a lot of current is being passed through a resistor and with it needing to be resistant to high heat and flame, cement resistors is a good design choice.
Typical power ratings range from 1W to 20W or more. Tolerances from the stated resistance value are around 5 percent.
Thermistors:
A thermistor is a thermally sensitive resistor whose resistance value changes with changes in operating temperature. Because of the self-heating effect of current in a thermistor, the device changes resistance with changes in current.
Thermistors exhibit either a positive temperature coefficient (PTC) or a negative temperature coefficient (NTC). If a thermistor has a positive temperature coefficient, its resistance increases as the operating temperature increases. Conversely, if a thermistor has a negative temperature coefficient, its resistance decreases as the operating temperature increases.
How much the resistance changes with changes in the operating temperature depends on the size and construction of the thermistor. It's always best to check the datasheet of the thermistor in use to find out all the specifications of the thermistors.
Thermistors are frequently used in electronic circuits that handle temperature measurement, temperature control, and temperature compensation.
Photoresistors:
Photoresistors are resistors whose resistance values change according to the light striking the surface of the resistor. In a dark environment, the resistance of a photoresistor is very high, possibly several MΩ, depending on the resistance rating of the specific photoresistor in use. When intense light hits the surface, the resistance of the photoresistor drops dramatically, possibly to as low as 400Ω.
Thus, photoresistors are variable resistors whose resistance values change in regard to the amount of light hitting its surface.
Leaded and non-leaded resistor types
Leaded resistors: This type of resistor has been used since the very first electronic components have been in use. Typically components were connected to terminal posts of one form or another and leads from the resistor element were needed. As time progressed, printed circuit boards were used, and the leads were inserted through holes in the boards and typically soldered on the reverse side where the tracks were to be found.
Surface mount resistors:
These resistor types have been used increasingly since the introduction of surface mount technology. Typically this type of resistor is manufactured using thin film technology. A full range of values can be obtained.
Step 4: Standard or Common Resistor Values
The Electronic Industries Association (EIA), and other authorities, specify standard values for resistors, sometimes referred to as the "preferred value" system. The preferred value system has its origins in the early years of the last century at a time when most resistors were carbon-graphite with relatively poor manufacturing tolerances. The rationale is simple - select values for components based on the tolerances with which they are able to be manufactured. Using 10% tolerance devices as an example, suppose that the first preferred value is 100 ohms. It makes little sense to produce a 105 ohm resistor since 105 ohms falls within the 10% tolerance range of the 100 ohm resistor. The next reasonable value is 120 ohms because the 100 ohm resistor with a 10% tolerance is expected to have a value somewhere between 900 and 110 ohms. The 120 ohm resistor has a value ranging between 110 and 130 ohms. Following this logic, the preferred values for 10% tolerance resistors between 100 and 1,000 ohms would be 100, 120, 150, 180, 220, 270, 330 and so on (rounded appropriately); this is the E12 series shown in the table below. The EIA "E" series specify the preferred values for various tolerances. The number following the "E" specifies the number of logarithmic steps per decade. The table below is normalized for the decade between 100 and 1,000. The values in any decade can be derived by merely dividing or multiplying the table entries by powers of 10. The series are as follows:
E6 20% tolerance,
E12 10% tolerance,
E24 5% tolerance (and usually 2% tolerance),
E48 2% tolerance,
E96 1% tolerance,
E192 .5, .25, .1% and higher tolerances.
Standard EIA Decade Resistor Values:
E6 series: (20% tolerance) 10, 15, 22, 33, 47, 68
E12 series: (10% tolerance) 10, 12, 15, 18, 22, 27, 33, 39, 47, 56, 68, 82
E24 series: (5% tolerance) 10, 11, 12, 13, 15, 16, 18, 20, 22, 24, 27, 30, 33, 36, 39, 43, 47, 51, 56, 62, 68, 75, 82, 91
E48 series: (2% tolerance) 100, 105, 110, 115, 121, 127, 133, 140, 147, 154, 162, 169, 178, 187, 196, 205, 215, 226, 237, 249, 261, 274, 287, 301, 316, 332, 348, 365, 383, 402, 422, 442, 464, 487, 511, 536, 562, 590, 619, 649, 681, 715, 750, 787, 825, 866, 909, 953
E96 series: (1% tolerance) 100, 102, 105, 107, 110, 113, 115, 118, 121, 124, 127, 130, 133, 137, 140, 143, 147, 150, 154, 158, 162, 165, 169, 174, 178, 182, 187, 191, 196, 200, 205, 210, 215, 221, 226, 232, 237, 243, 249, 255, 261, 267, 274, 280, 287, 294, 301, 309, 316, 324, 332, 340, 348, 357, 365, 374, 383, 392, 402, 412, 422, 432, 442, 453, 464, 475, 487, 491, 511, 523, 536, 549, 562, 576, 590, 604, 619, 634, 649, 665, 681, 698, 715, 732, 750, 768, 787, 806, 825, 845, 866, 887, 909, 931, 959, 976
E192 series: (0.5, 0.25, 0.1 and 0.05% tolerance) 100, 101, 102, 104, 105, 106, 107, 109, 110, 111, 113, 114, 115, 117, 118, 120, 121, 123, 124, 126, 127, 129, 130, 132, 133, 135, 137, 138, 140, 142, 143, 145, 147, 149, 150, 152, 154, 156, 158, 160, 162, 164, 165, 167, 169, 172, 174, 176, 178, 180, 182, 184, 187, 189, 191, 193, 196, 198, 200, 203, 205, 208, 210, 213, 215, 218, 221, 223, 226, 229, 232, 234, 237, 240, 243, 246, 249, 252, 255, 258, 261, 264, 267, 271, 274, 277, 280, 284, 287, 291, 294, 298, 301, 305, 309, 312, 316, 320, 324, 328, 332, 336, 340, 344, 348, 352, 357, 361, 365, 370, 374, 379, 383, 388, 392, 397, 402, 407, 412, 417, 422, 427, 432, 437, 442, 448, 453, 459, 464, 470, 475, 481, 487, 493, 499, 505, 511, 517, 523, 530, 536, 542, 549, 556, 562, 569, 576, 583, 590, 597, 604, 612, 619, 626, 634, 642, 649, 657, 665, 673, 681, 690, 698, 706, 715, 723, 732, 741, 750, 759, 768, 777, 787, 796, 806, 816, 825, 835, 845, 856, 866, 876, 887, 898, 909, 920, 931, 942, 953, 965, 976, 988
When designing equipment, it is good practice to keep to the lowest E-series section, i.e. it is better to use E6 rather than E12. In this way the number of different parts in any equipment can be minimised. If decade values, i.e. 100R, 1K, 10, etc can be used so much the better. These are very common resistor values and ore widely used. It also reduces the variety of components and makes inventory more manageable.
For many digital designs where the resistor is used as a pull up or pull down, the resistor value is of little consequence and this is easy. For analogue designs it is a little more complicated, and E12, or E24 values are needed. E48, E96 or even E192 series values are needed for high accuracy and close tolerance requirements. As the higher order series are used less, their costs are also normally higher. Using common resistor values can reduce costs as well as reducing inventory.
Step 5: Resistor Color Codes
We know many different types of Resistor available and that they can be used in both electrical and electronic circuits to control the flow of current or to produce a voltage in many different ways. But in order to do this the actual resistor needs to have some form of “resistive” or “resistance” value. Resistors are available in a range of different resistance values from fractions of an Ohm ( Ω ) to millions of Ohms.
The resistance value, tolerance, and wattage rating are generally printed onto the body of the resistor as numbers or letters when the resistors body is big enough to read the print, such as large power resistors. But when the resistor is small such as a 1/4W carbon or film type, these specifications must be shown in some other manner as the print would be too small to read.
So to overcome this, small resistors use coloured painted bands to indicate both their resistive value and their tolerance with the physical size of the resistor indicating its wattage rating. These coloured painted bands produce a system of identification generally known as a Resistors Colour Code. An international and universally accepted Resistor Colour Code Scheme was developed many years ago as a simple and quick way of identifying a resistors ohmic value no matter what its size or condition. It consists of a set of individual coloured rings or bands in spectral order representing each digit of the resistors value. The resistor colour code markings are always read one band at a time starting from the left to the right, with the larger width tolerance band oriented to the right side indicating its tolerance. By matching the colour of the first band with its associated number in the digit column of the colour chart below the first digit is identified and this represents the first digit of the resistance value. Again, by matching the colour of the second band with its associated number in the digit column of the colour chart we get the second digit of the resistance value and so on. Then the resistor colour code is read from left to right as illustrated in figure.
Tips for reading resistor codes
The reading direction might not always be clear. Sometimes the increased space between band 3 and 4 give away the reading direction. Also, the first band is usually the closest to a lead. A gold or silver band (the tolerance) is always the last band.It is a good practice to check the manufacturer’s documentation to be sure about the used coding system. Even better is to measure the resistance with a multi-meter. In some cases this might even be the only way to figure out the resistance; for example when the color bands are burnt off.
Surface Mount Resistors
Surface Mount Resistors or SMD Resistors, are very small rectangular shaped metal oxide film resistors designed to be soldered directly onto the surface, hence their name, of a circuit board. Surface mount resistors generally have a ceramic substrate body onto which is deposited a thick layer of metal oxide resistance. The resistive value of the resistor is controlled by increasing the desired thickness, length or type of deposited film being used and highly accurate low tolerance resistors, down to 0.1% can be produced. They also have metal terminals or caps at either end of the body which allows them to be soldered directly onto printed circuit boards. Surface Mount Resistors are printed with either a 3 or 4-digit numerical code which is similar to that used on the more common axial type resistors to denote their resistive value. Standard SMD resistors are marked with a three-digit code, in which the first two digits represent the first two numbers of the resistance value with the third digit being the multiplier, either x1, x10, x100 etc. For example:
“103” = 10 × 1,000 ohms = 10 kiloΩ´s
“392” = 39 × 100 ohms = 3.9 kiloΩ´s
“563” = 56 × 1,000 ohms = 56 kiloΩ´s
“105” = 10 × 100,000 ohms = 1 MegaΩ
Surface mount resistors that have a value of less than 100Ω’s are usually written as: “390”,“470”, “560” with the final zero representing a 10^0 multiplier, which is equivalent to 1. For example: “390” = 39 × 1Ω = 39Ω´s or 39RΩ “470” = 47 × 1Ω = 47Ω´s or 47RΩ Resistance values below ten have a letter “R” to denote the position of the decimal point, so that 4R7 = 4.7Ω. Surface mount resistors that have a “000” or “0000” markings are zero-Ohm (0Ω) resistors or in other words shorting links, since these components have zero resistance.
Step 6: Series Parallel Resistor
Resistors are paired together all the time in electronics, usually in either a series or parallel circuit. When resistors are combined in series or parallel, they create a total resistance, which can be calculated using one of two equations. Knowing how resistor values combine comes in handy if you need to create a specific resistor value.
Series resistors
When connected in series resistor values simply add up. So, for example, if you just have to have a 12.33kΩ resistor, seek out some of the more common resistor values of 12kΩ and 330Ω, and butt them up together in series.
Parallel resistors
Finding the resistance of resistors in parallel isn’t quite so easy. The total resistance of N resistors in parallel is the inverse of the sum of all inverse resistances. This equation might make more sense than that last sentence.
Some example applications of resistors
Current Limiter
One main use of resistor as a current limiter. Resistors are key in making sure LEDs don’t blow up when power is applied. By connecting a resistor in series with an LED, current flowing through the two components can be limited to a safe value. Note the circuit given below. Resistor R is connected in series to the LED.
For calculating the value of current limiting resistor for led two important thing should be consider, the typical forward voltage (Vf), and the maximum forward current (If). The typical forward voltage is the voltage which is required to make an LED light up, and it varies (usually somewhere between 1.7V and 3.4V) depending upon the color of the LED. The maximum forward current is usually around 20mA for basic LEDs; continuous current through the LED should always be equal to or less than that current rating. Once you got the value of Vf and If the the size of the current limiting resistor can be calculated using the formula:
R = (Vs - Vf) / If
where, Vs is the supply voltage. For our case suppose we power it from 5V source and led forward voltage is 1.8 V. Then value of resistor for 10mA led current:
R = (5 - 1.8) / 10 = 320 ohm.
Voltage Dividers
A voltage divider is a resistor circuit which turns a large voltage into a smaller one. Using just two resistors in series, an output voltage can be created that’s a fraction of the input voltage and depends on the ratio of the two resistors.
In the circuit on right side two resistors, R1 and R2, are connected in series and a voltage source (Vin) is connected across them. The voltage from Vout to GND can be calculated as:
Vout = Vin x R2 / (R1 + R2)
For example, if R1 was 1.7kΩ and R2 was 3.3kΩ, a 5V input voltage could be turned into 3.3V at the Vout terminal.
Voltage dividers are very handy for reading resistive sensors, like photocells, flex sensors, and force-sensitive resistors. One half of the voltage divider is the sensor, and the part is a static resistor. The output voltage between the two components is connected to an analog-to-digital converter on a microcontroller (MCU) to read the sensor’s value.
Pull-up Resistors
A pull-up resistor is used when you need to bias a microcontroller’s input pin to a known state. One end of the resistor is connected to the MCU’s pin, and the other end is connected to a high voltage (usually 5V or 3.3V).
Without a pull-up resistor, inputs on the MCU could be left floating. There’s no guarantee that a floating pin is either high (5V) or low (0V).
Pull-up resistors are often used when interfacing with a button or switch input. The pull-up resistor can bias the input-pin when the switch is open. And it will protect the circuit from a short when the switch is closed.
In the circuit above, when the switch is open the MCU’s input pin is connected through the resistor to 5V. When the switch closes, the input pin is connected directly to GND.
The value of a pull-up resistor doesn’t usually need to be anything specific. But it should be high enough that not too much power is lost if 5V or so is applied across it. Usually values around 10kΩ work well.
Step 7: Capacitor
A capacitor is a bit like a battery, but it has a different job to do. A battery uses chemicals to store electrical energy and release it very slowly through a circuit; sometimes (in the case of a quartz watch) it can take several years. A capacitor generally releases its energy much more rapidly—often in seconds or less. If you're taking a flash photograph, for example, you need your camera to produce a huge burst of light in a fraction of a second. A capacitor attached to the flash gun charges up for a few seconds using energy from your camera's batteries. (It takes time to charge a capacitor and that's why you typically have to wait a little while.) Once the capacitor is fully charged, it can release all that energy in an instant through the xenon flash bulb. Zap!
There are many different kinds of capacitors available from very small capacitor beads used in resonance circuits to large power factor correction capacitors, but they all do the same thing, they store charge. In its basic form, a Capacitor consists of two or more parallel conductive (metal) plates which are not connected or touching each other, but are electrically separated either by air or by some form of a good insulating material such as waxed paper, mica, ceramic, plastic or some form of a liquid gel as used in electrolytic capacitors. The insulating layer between a capacitors plates is commonly called the Dielectric. A Typical Capacitor
Due to this insulating layer, DC current can not flow through the capacitor as it blocks it allowing instead a voltage to be present across the plates in the form of an electrical charge.
Capacitors and capacitance
The amount of electrical energy a capacitor can store is called its capacitance. The capacitance of a capacitor is a bit like the size of a bucket: the bigger the bucket, the more water it can store; the bigger the capacitance, the more electricity a capacitor can store. There are three ways to increase the capacitance of a capacitor. One is to increase the size of the plates. Another is to move the plates closer together. The third way is to make the dielectric as good an insulator as possible. Capacitors use dielectrics made from all sorts of materials. In transistor radios, the tuning is carried out by a largevariable capacitor that has nothing but air between its plates. In most electronic circuits, the capacitors are sealed components with dielectrics made of ceramics such as mica and glass, paper soaked in oil, or plastics such as mylar.
The size of a capacitor is measured in units called farads (F), named for English electrical pioneer Michael Faraday (1791–1867). One farad is a huge amount of capacitance so, in practice, most of the capacitors we come across are just fractions of a farad—typically microfarads (millionths of a farad, written μF), nanofarads (thousand-millionths of a farad written nF), and picofarads (million millionths of a farad, written pF).Supercapacitors store far bigger charges, sometimes rated in thousands of farads.
Types of Capacitors
There are many different types of capacitors and they each vary in their characteristics and each have their own advantages and disadvantages.
Some types of capacitors can charge up to higher voltages and, thus, can be used in high voltage applications. Some capacitors can charge up to very high charges, such as aluminum electrolytic capacitors. Some capacitors have very low leakage low leakage rates and others have very high leakage rates. All of these factors determine how and in what application each of the capacitors will be used in circuits.
Based on the design, capacitors are categorized in these different types:
Electrolytic type:
For most of applications we use Electrolytic type Capacitors. They are very important for an electronic student as they are easy to get and to use, and they are inexpensive too.
Electrolytic Capacitors are generally used when very large capacitance values are required typically above 1μF. Here instead of using a very thin metallic film layer for one of the electrodes, a semi-liquid electrolyte solution in the form of a jelly or paste is used which serves as the second electrode (usually the cathode).
The dielectric is a very thin layer of oxide which is grown electro-chemically in production with the thickness of the film being less than ten microns. This insulating layer is so thin that it is possible to make capacitors with a large value of capacitance for a small physical size as the distance between the plates, d is very small.
The majority of electrolytic types of capacitors are Polarised, that is the DC voltage applied to the capacitor terminals must be of the correct polarity, i.e. positive to the positive terminal and negative to the negative terminal as an incorrect polarisation will break down the insulating oxide layer and permanent damage may result. All polarised electrolytic capacitors have their polarity clearly marked with a negative sign to indicate the negative terminal and this polarity must be followed. Electrolytic Capacitors are generally used in DC power supply circuits due to their large capacitance’s and small size to help reduce the ripple voltage or for coupling and decoupling applications. One main disadvantage of electrolytic capacitors is their relatively low voltage rating and due to the polarisation of electrolytic capacitors, it follows then that they must not be used on AC supplies. Electrolytic’s generally come in two basic forms; Aluminium Electrolytic Capacitors and Tantalum Electrolytic Capacitors.
An electrolytic capacitor is usually labeled with these things:
1. Capacitance value.
2. Maximum voltage.
3. Maximum temperature.
4. Polarity.
For an electrolytic capacitor, the capacitance is measured in micro Farad. Based on requirement the appropriate capacitor is chosen. With higher capacitance, the size of capacitor also increases.
Voltage Rating of a Capacitor
All capacitors have a maximum voltage rating and when selecting a capacitor consideration must be given to the amount of voltage to be applied across the capacitor. The maximum amount of voltage that can be applied to the capacitor without damage to its dielectric material is generally given in the data sheets as: WV, (working voltage) or as WV DC, (DC working voltage). If the voltage applied across the capacitor becomes too great, the dielectric will break down (known as electrical breakdown) and arcing will occur between the capacitor plates resulting in a short-circuit. The working voltage of the capacitor depends on the type of dielectric material being used and its thickness. The DC working voltage of a capacitor is just that, the maximum DC voltage and NOT the maximum AC voltage as a capacitor with a DC voltage rating of 100 volts DC cannot be safely subjected to an alternating voltage of 100 volts. Since an alternating voltage has an r.m.s. value of 100 volts but a peak value of over 141 volts!. Then a capacitor which is required to operate at 100 volts AC should have a working voltage of at least 200 volts. In practice, a capacitor should be selected so that its working voltage either DC or AC should be at least 50 percent greater than the highest effective voltage to be applied to it.
Polyester type:
Polyester capacitors are capacitors composed of metal plates with polyester film between them, or a metallised film is deposited on the insulator.
Polyester capacitors are available in the range of 1nF to 15µF, and with working voltages from 50V to 1500V. They come with the tolerance ranges of 5%, 10%, and 20%. They have a high temperature coefficient. They have high isolation resistance, so they are good choice capacitors for coupling and/or storage applications. Compared with most other types, polyester capacitors have high capacitance per unit volume. This means more capacitance can fit into a physically smaller capacitor. This feature, together with their relatively low price makes polyester capacitors a widely used, popular, and cheap capacitor.
Tantalum type:
Tantalum Capacitors are capacitors that are made of tantalum pentoxide. Tantalum capacitors, just like aluminum, are electrolytic capacitors, which means they are polarized. Their main advantages (especially over aluminum capacitors) is that they are smaller, lighter, and more stable. They have lower leakage rates and less inductance between leads. However, their disadvantags are they have a lower maximum capacitance storage and lower maximum working voltage. They are also more prone to damage from high current spikes. For the last reason, tantalum capacitors are used mostly in analog signal systems that lack high current-spike noise.
Ceramic Capacitors:
Ceramic Capacitors or Disc Capacitors as they are generally called, are made by coating two sides of a small porcelain or ceramic disc with silver and are then stacked together to make a capacitor. For very low capacitance values a single ceramic disc of about 3-6mm is used. Ceramic capacitors have a high dielectric constant (High-K) and are available so that relatively high capacitance’s can be obtained in a small physical size. Ceramic Capacitor
They exhibit large non-linear changes in capacitance against temperature and as a result are used as de-coupling or by-pass capacitors as they are also non-polarized devices. Ceramic capacitors have values ranging from a few picofarads to one or two microfarads, ( μF ) but their voltage ratings are generally quite low. Ceramic types of capacitors generally have a 3-digit code printed onto their body to identify their capacitance value in pico-farads. Generally the first two digits indicate the capacitors value and the third digit indicates the number of zero’s to be added. For example, a ceramic disc capacitor with the markings 103 would indicate 10 and 3 zero’s in pico-farads which is equivalent to 10,000 pF or 10nF. Likewise, the digits 104 would indicate 10 and 4 zero’s in pico-farads which is equivalent to 100,000 pF or 100nF and so on. So on the image of the ceramic capacitor above the numbers 154 indicate 15 and 4 zero’s in pico-farads which is equivalent to 150,000 pF or 150nF or 0.15uF. Letter codes are sometimes used to indicate their tolerance value such as: J = 5%, K = 10% or M = 20% etc.
General uses of Capacitors
- Smoothing, especially in power supply applications which required converting the signal from AC to DC.
- Storing Energy.
- Signal decoupling and coupling as a capacitor coupling that blocks DC current and allow AC current to pass in circuits.
- Tuning, as in radio systems by connecting them to LC oscillator and for tuning to the desired frequency.
- Timing, due to the fixed charging and discharging time of capacitors.
- For electrical power factor correction and many more applications.
Step 8: Inductor
An inductor is a passive electronic component that stores energy in the form of a magnetic field. As we know resistor resists the flow of current, inductor resists the change in flowing current through it. So for dc current inductor is nothing but like a conductor. In other words, inductors resist or oppose changes of current but will easily pass a steady state DC current.
The current, that flows through an inductor produces a magnetic flux that is proportional to it. But unlike a Capacitor which oppose a change of voltage across their plates, an inductor opposes the rate of change of current flowing through it due to the build up of self-induced energy within its magnetic field.
In its most basic form, an Inductor is nothing more than a coil of wire wound around a central core. For most coils the current, flowing through the coil produces a magnetic flux around it that is proportional to this flow of electrical current.
The Inductor, also called a choke. Inductors are formed with wire tightly wrapped around a solid central core which can be either a straight cylindrical rod or a continuous loop or ring to concentrate their magnetic flux. The schematic symbol for a inductor is that of a coil of wire so therefore, a coil of wire can also be called an Inductor. Inductors usually are categorised according to the type of inner core they are wound around, for example, hollow core (free air), solid iron core or soft ferrite core with the different core types being distinguished by adding continuous or dotted parallel lines next to the wire coil as shown below.
The standard unit of inductance is the henry, abbreviated H. This is a large unit. More common units are the microhenry, abbreviated µH (1 µH =10^-6H) and the millihenry, abbreviated mH (1 mH =10^-3 H). Occasionally, the nanohenry (nH) is used (1 nH = 10^-9 H).
Applications of Inductors
Filters
Inductors are used extensively with capacitors and resistors to create filters for analog circuits and in signal processing. Alone, an inductor functions as a low-pass filter, since the impedance of an inductor increases as the frequency of a signal increases. When combined with a capacitor, whose impedance decreases as the frequency of a signal increase, a notched filter can be made that only allows a certain frequency range to pass through. By combining capacitors, inductors, and resistors in a number of ways advanced filter topologies can be created for any number of applications. Filters are used in most electronics, although capacitors are often used rather than inductors when possible since they are smaller and cheaper.
Sensors
Contactless sensors are prized for their reliability and ease of operation and inductors can be used to sense magnetic fields or the presence of magnetically permeable material from a distance. Inductive sensors are used at nearly every intersection with a traffic light to detect the amount of traffic and adjust the signal accordingly. These sensors work exceptionally well for cars and trucks, but some motorcycles and other vehicles do not have enough of a signature to be detected by the sensors without a little extra boost by adding a h3 magnet to the bottom of the vehicle. Inductive sensors are limited in two major ways, either the object to be sensed must be magnetic and induce a current in the sensor or the sensor must be powered to detect the presence of materials that interact with a magnetic field. This limits the applications of inductive sensors and has a major impact on designs that use them.
Transformers
Combining inductors that have a shared magnetic path will form a transformer. The transformer is a fundamental component of national electrical grids and found in many power supplies as well to increase or decrease voltages to a desired level. Since magnetic fields are created by a change in current, the faster the current changes (increase in frequency) the more effective a transformer operates. Of course, as the frequency of the input increases, the impedance of the inductor begins to limit the effectiveness of a transformer.
Motors
Normally inductors are in a fixed position and not allowed to move to align themselves with any nearby magnetic field. Inductive motor leverage the magnetic force applied to inductors to turn electrical energy in to mechanical energy. Inductive motors are designed so that a rotating magnetic field is created in time with an AC input. Since the speed of rotation is controlled by the input frequency, induction motors are often used in fixed speed applications that can be powered directly from 50/60hz mains power. The biggest advantage of inductive motors over other designs is that no electrical contact is required between the rotor and the motor which makes inductive motors very robust and reliable.
Energy Storage
Like capacitors, inductors can be used for energy storage. Unlike capacitors, inductors have a severe limitation on how long they can store energy since the energy is stored in a magnetic field which collapses quickly once power is removed. The main use for inductors as energy storage is in switch-mode power supplies, like the power supply in a PC. In the simpler, non-isolated switch-mode power supplies, a single inductor is used in place of transformer and energy storage component. In these circuits, the ratio of the time the inductor is powered to the time it is unpowered determines the input to output voltage ratio.
Inductors are also used for wireless power transfer and in electro-mechanical relay.
Step 9: Diode
A diode is a specialized electronic component with two electrodes called the anode and the cathode. Most diodes are made with semiconductor materials such as silicon, germanium, or selenium. Diodes can be used as rectifiers, signal limiters, voltage regulators, switches, signal modulators, signal mixers, signal demodulators, and oscillators.
The fundamental property of a diode is its tendency to conduct electric current in only one direction. When the cathode is negatively charged relative to the anode at a voltage greater than a certain minimum called forward breakover, then current flows through the diode. If the cathode is positive with respect to the anode, is at the same voltage as the anode, or is negative by an amount less than the forward breakover voltage, then the diode does not conduct current. This is a simplistic view, but is true for diodes operating as rectifiers, switches, and limiters. The forward breakover voltage is approximately six tenths of a volt (0.6 V) for silicon devices, 0.3 V for germanium devices, and 1 V for selenium devices.
Breakdown Voltage
If a large enough negative voltage is applied to the diode, it will give in and allow current to flow in the reverse direction. This large negative voltage is called the breakdown voltage. Some diodes are actually designed to operate in the breakdown region, but for most normal diodes it’s not very healthy for them to be subjected to large negative voltages. For normal diodes this breakdown voltage is around -50V to -100V, or even more negative.
Types of Diodes
Many different types of diodes today are in use in electronics. The different kinds each have their own specialized uses. I will only discuss about more common types.
Rectifier Diode:
These diodes are used to rectify alternating power inputs in power supplies. A rectifier or power diode is a standard diode with a much higher maximum current rating. This higher current rating usually comes at the cost of a larger forward voltage. The 1N4001, for example, has a current rating of 1A and a forward voltage of 1.1V.
Signal diodes:
A small signal diode is a small non-linear semiconductor which is often used in electronic circuits where high frequencies or small currents are involved in television, radio and digital logic circuits. Small signal diodes are smaller in size compared to regular power diodes. They usually have a medium-high forward voltage drop and a low maximum current rating. A common example of a signal diode is the1N4148. Very general purpose, it’s got a typical forward voltage drop of 0.72V and a 300mA maximum forward current rating.
Schottky Diodes:
These diodes feature lower forward voltage drop as compared to the ordinary silicon PN junction diodes. The voltage drop may be somewhere between 0.15 and 0.4 volts at low currents, as compared to the 0.6 volts for a silicon diode. In order to achieve this performance, these diodes are constructed differently from normal diodes, with metal to semiconductor contact. Schottky diodes are used in RF applications, rectifier applications and clamping diodes.
Zener diodes:
Zener diodes are the weird outcast of the diode family. They’re usually used to intentionally conduct reverse current. Zener’s are designed to have a very precise breakdown voltage, called the zener breakdown or zener voltage. When enough current runs in reverse through the zener, the voltage drop across it will hold steady at the breakdown voltage. Taking advantage of their breakdown property, Zener diodes are often used to create a known reference voltage at exactly their Zener voltage. They can be used as a voltage regulator for small loads, but they’re not really made to regulate voltage to circuits that will pull significant amounts of current.
Light-Emitting Diodes:
Like normal diodes, LEDs only allow current through one direction. They also have a forward voltage rating, which is the voltage required for them to light up. The VF rating of an LED is usually larger than that of a normal diode (1.2~3V), and it depends on the color the LED emits. For example, the rated forward voltage of a Super Bright Blue LED is around 3.3V, while that of the equal size Super Bright Red LED is only 2.2V. I will discuss about LEDs more detail later.
Photodiode:
Photodiodes are used to detect light and feature wide, transparent junctions. Generally, these diodes operate in reverse bias, wherein even small amounts of current flow, resulting from the light, can be detected with ease. Photodiodes can also be used to generate electricity, used as solar cells and even in photometry.
Laser Diode:
This type of diode is different from the LED type, as it produces coherent light. These diodes find their application in DVD and CD drives, laser pointers, etc. Laser diodes are more expensive than LEDs. However, they are cheaper than other forms of laser generators. Moreover, these laser diodes have limited life.
Step 10: LED
Light emitting diodes, commonly called LEDs, are real unsung heroes in the electronics world. They do dozens of different jobs and are found in all kinds of devices. Among other things, they form numbers on digital clocks, transmit information from remote controls, light up watches and tell you when your appliances are turned on. Collected together, they can form images on a jumbo television screen or illuminate a traffic light.
Basically, LEDs are just tiny light bulbs that fit easily into an electrical circuit. But unlike ordinary incandescent bulbs, they don't have a filament that will burn out, and they don't get especially hot. They are illuminated solely by the movement of electrons in a semiconductor material, and they last just as long as a standard transistor. The lifespan of an LED surpasses the short life of an incandescent bulb by thousands of hours. Tiny LEDs are already replacing the tubes that light up LCD HDTVs to make dramatically thinner televisions.
LEDs are mostly used for two things: illumination and indication. Illumination means to "shine light onto something" - like a flashlight or headlights. You want your headlights to be bright as heck. Indication mean to "point something out" - like a turn signal or brake lights on a car. You don't want your car's turn signal to blind people! Diffused LEDs are really good at indication, they look soft and uniform and you can see them well from any angle. Clear LEDs are really good at illumination, the light is direct and powerful - but you can't see them well from an angle because the light is only going forward.
LED circuit design
LED's are diodes, which are biased with a current rather than voltage. Simply, when LED's are "fed" with some current in the forward direction (plus to minus, or anode to cathode it would start to emit light at some minimum current. A typical red LED's require about 10mA to 20mA current for decent brightness. Any more may not help much--LED's would be stressed when pushed beyond the limits and may be destroyed.
Since LED's are current devices, a voltage can not be applied directly across it, LED's cannot be connected directly to the battery or power supply. The LED will be instantly destroyed because the current is too great. The current must be reduced. The easiest way to do this is by using a resistor. The resistor will lower the current and drop the voltage down to a manageable level.
So, how do we figure out what value resistor to use? We shall use the ohm's law for this. Ohms law states that voltage is the product of the current and the resistance, or V = IR, where "I" is the current.
Calculate the LED resistor value with the following formula:
LED Resistor Value, R = (supply voltage - LED voltage) / LED current
In our example:
Say we use a 9V battery, then supply voltage = 9V. LED voltage for red LED's, from Step 2 is 2.0 V LED current is 20 mA (this is a typical value if not provided by the manufacturer) If the resistor value is not available, then choose the nearest standard resistor value which is greater. If you want to increase the battery life you can select a higher resistor value to reduce current. The reduced current will result in a dimmer LED. For 15mA led current, R = (9 - 2.0) / 15 mA = 466 ohms, use the next higher standard value = 470 ohms.
Step 11: Transistor
Transistors can be regarded as a type of electronic switch, as can many electronic components. Transistor is much more faster than mechanical switch.
There are two types of basic transistor out there: bi-polar junction (BJT) and metal-oxide field-effect (MOSFET), and there are actually two versions of the BJT: NPN and PNP. Most circuits tend to use NPN. There are hundreds of transistors which work at different voltages but all of them fall into these two categories. Transistors are manufactured in different shapes but they have three leads (legs). The BASE - which is the lead responsible for activating the transistor.The COLLECTOR - which is the positive lead.The EMITTER - which is the negative lead.
A transistor is really simple—and really complex. Let's start with the simple part. A transistor is a miniature electronic component that can do two different jobs. It can work either as an amplifier or a switch:
When it works as an amplifier, it takes in a tiny electric current at one end (an input current) and produces a much bigger electric current (an output current) at the other. In other words, it's a kind of current booster. That comes in really useful in things like hearing aids, one of the first things people used transistors for. A hearing aid has a tiny microphone in it that picks up sounds from the world around you and turns them into fluctuating electric currents. These are fed into a transistor that boosts them and powers a tiny loudspeaker, so you hear a much louder version of the sounds around you.
Transistors can also work as switches. A tiny electric current flowing through one part of a transistor can make a much bigger current flow through another part of it. In other words, the small current switches on the larger one. This is essentially how all computer chips work. For example, a memory chip contains hundreds of millions or even billions of transistors, each of which can be switched on or off individually. Since each transistor can be in two distinct states, it can store two different numbers, zero and one. With billions of transistors, a chip can store billions of zeros and ones, and almost as many ordinary numbers and letters (or characters, as we call them). More about this in a moment.
Operation Modes
Unlike resistors, which enforce a linear relationship between voltage and current, transistors are non-linear devices. They have four distinct modes of operation, which describe the current flowing through them. (When we talk about current flow through a transistor, we usually mean current flowing from collector to emitter of an NPN transistor. The four transistor operation modes are:
Saturation – The transistor acts like a short circuit. Current freely flows from collector to emitter.
Cut-off – The transistor acts like an open circuit. No current flows from collector to emitter.
Active – The current from collector to emitter is proportional to the current flowing into the base.
Reverse-Active – Like active mode, the current is proportional to the base current, but it flows in reverse.
Applications: Switches
One of the most fundamental applications of a transistor is using it to control the flow of power to another part of the circuit – using it as an electric switch. Driving it in either cutoff or saturation mode, the transistor can create the binary on/off effect of a switch. Transistor switches are critical circuit-building blocks; they’re used to make logic gates, which go on to create microcontrollers, microprocessors, and other integrated circuits.
Transistor Switch
Let’s look at the most fundamental transistor-switch circuit: an NPN switch. Here we use an NPN to control a high-power LED.
Our control input flows into the base, the output is tied to the collector, and the emitter is kept at a fixed voltage.
While a normal switch would require an actuator to be physically flipped, this switch is controlled by the voltage at the base pin. A microcontroller I/O pin, like those on an Arduino, can be programmed to go high or low to turn the LED on or off.
When the voltage at the base is greater than 0.6V (or whatever your transistor’s Vth might be), the transistor starts saturating and looks like a short circuit between collector and emitter. When the voltage at the base is less than 0.6V the transistor is in cutoff mode – no current flows because it looks like an open circuit between C and E.
The circuit above is called a low-side switch, because the switch – our transistor – is on the low (ground) side of the circuit. Alternatively, we can use a PNP transistor to create a high-side switch:
Similar to the NPN circuit, the base is our input, and the emitter is tied to a constant voltage. This time however, the emitter is tied high, and the load is connected to the transistor on the ground side.
This circuit works just as well as the NPN-based switch, but there’s one huge difference: to turn the load “on” the base must be low. This can cause complications, especially if the load’s high voltage (VCC in this picture) is higher than our control input’s high voltage. For example, this circuit wouldn’t work if you were trying to use a 5V-operating Arduino to switch on a 12V motor. In that case it’d be impossible to turn the switch off because VB would always be less than VE.
Base Resistors
You’ll notice that each of those circuits uses a series resistor between the control input and the base of the transistor. Don’t forget to add this resistor! A transistor without a resistor on the base is like an LED with no current-limiting resistor.
Recall that, in a way, a transistor is just a pair of interconnected diodes. We’re forward-biasing the base-emitter diode to turn the load on. The diode only needs 0.6V to turn on, more voltage than that means more current. Some transistors may only be rated for a maximum of 10-100mA of current to flow through them. If you supply a current over the maximum rating, the transistor might blow up.
The series resistor between our control source and the base limits current into the base. The base-emitter node can get its happy voltage drop of 0.6V, and the resistor can drop the remaining voltage. The value of the resistor, and voltage across it, will set the current.
The resistor needs to be large enough to effectively limit the current, but small enough to feed the base enough current. 1mA to 10mA will usually be enough and base resistor value may be 1k to 10k, but check your transistor’s datasheet to make sure.
Some common BJTs frequently used in hobbyist project
Name | Type | Vce | Ic | Pd | ft |
---|---|---|---|---|---|
2N2222 | NPN | 40V | 800mA | 625mW | 300MHz |
BC548 | NPN | 30V | 100mA | 500mW | 300MHz |
2N3904 | NPN | 40V | 200mA | 625mW | 270MHz |
2N3906 | PNP | -40V | -200mA | 625mW | 250MHz |
BC557 | PNP | -45V | -100mA | 500mW | 150MHz |
TIP120 (power) | NPN | 60V | 5A | 65W | - |
Please check the datasheet for details.
MOSFET
The metal–oxide–semiconductor field-effect transistor (MOSFET, MOS-FET, or MOS FET) is another type of transistor used for amplifying or switching electronic signals.
The main advantage of a MOSFET over a regular transistor is that it requires very little current to turn on (less than 1mA), while delivering a much higher current to a load (10 to 50A or more).
The Metal Oxide Semiconductor Field Effect Transistor, or MOSFET for short, has an extremely high input gate resistance with the current flowing through the channel between the source and drain being controlled by the gate voltage. Because of this high input impedance and gain, MOSFETs can be easily damaged by static electricity if not carefully protected or handled.
MOSFET’s are ideal for use as electronic switches or as common-source amplifiers as their power consumption is very small. Typical applications for metal oxide semiconductor field effect transistors are in Microprocessors, Memories, Calculators and Logic CMOS Gates etc.
Step 12: Voltage Regulators
A voltage regulator generates a fixed output voltage of a preset magnitude that remains constant regardless of changes to its input voltage or load conditions. There are two types of voltage regulators:
- Linear
- Switching
The linear regulator's power dissipation is directly proportional to its output current for a given input and output voltage, so typical efficiencies can be 50% or even lower. Using the optimum components, a switching regulator can achieve efficiencies in the 90% range. However, the noise output from a linear regulator is much lower than a switching regulator with the same output voltage and current requirements. Typically, the switching regulator can drive higher current loads than a linear regulator.
Linear regulator
A linear regulator employs an active (BJT or MOSFET) pass device (series or shunt) controlled by a high gain differential amplifier. It compares the output voltage with a precise reference voltage and adjusts the pass device to maintain a constant output voltage.
This regulating device acts like a variable resistor and continuously adjusts the voltage divider network in order to maintain an output voltage which is constant. The difference between the input voltage and regulated voltage is continually dissipating as waste heat. Due to linear voltage regulators being often used in several electronic devices, linear regulators in integrated circuit (IC) form are very common. There are several different kinds of linear regulators.
All linear regulators require an input voltage at least some minimum amount higher than the desired output voltage. That minimum amount is called the dropout voltage. For example, a common regulator such as the 7805 has an output voltage of 5V, but can only maintain this if the input voltage remains above about 7V, before the output voltage begins sagging below the rated output. Its dropout voltage is therefore 7V − 5V = 2V. There are two types of linear regulator:
Fixed regulators
"Fixed" three-terminal linear regulators are commonly available to generate fixed voltages of plus 3 V, and plus or minus 5 V, 6V, 9 V, 12 V, or 15 V, when the load is less than 1.5 A. The "78xx" series (7805, 7812, etc.) regulate positive voltages while the "79xx" series (7905, 7912, etc.) regulate negative voltages. Often, the last two digits of the device number are the output voltage (e.g., a 7805 is a +5 V regulator, while a 7915 is a −15 V regulator). There are variants on the 78xx series ICs, such as 78L and 78S, some of which can supply up to 2 Amps.
Variable regulators
An adjustable regulator generates a fixed low nominal voltage between its output and its adjust terminal (equivalent to the ground terminal in a fixed regulator). This family of devices includes low power devices like LM723 and medium power devices like LM317 and L200. Some of the variable regulators are available in packages with more than three pins, including dual in-line packages. They offer the capability to adjust the output voltage by using external resistors of specific values.
The LM317 series (+1.25V) regulates positive voltages while the LM337 series (−1.25V) regulates negative voltages. The adjustment is performed by constructing a potential divider with its ends between the regulator output and ground, and its centre-tap connected to the 'adjust' terminal of the regulator. The ratio of resistances determines the output voltage using the same feedback mechanisms described earlier.
Commonly used linear voltage regulator
L7805 (Voltage Regulator - 5V): This is the basic L7805 voltage regulator, a three-terminal positive regulator with a 5V fixed output voltage. This fixed regulator provides a local regulation, internal current limiting, thermal shut-down control, and safe area protection for your project. Each one of these voltage regulators can output a max current of 1.5A.
L7812 (Voltage Regulator - 12V): This is the basic L7812 voltage regulator, a three-terminal positive regulator with a 12V fixed output voltage. This fixed regulator provides a local regulation, internal current limiting, thermal shut-down control, and safe area protection for your project. Each one of these voltage regulators can output a max current of 1.5A.
LM317 (Adjustable 1.25V to 37V): TheLM317 device is an adjustable three-terminal positive-voltage regulator capable of supplying more than1.5 A over an output-voltage range of 1.25 V to 37V. It requires only two external resistors to set the output voltage. The device features a typical line regulation of 0.01% and typical load regulation of 0.1%. It includes current limiting, thermal overload protection, and safe operating area protection.
Please check datasheet for details.
Switching regulator
A switching regulator converts the dc input voltage to a switched voltage applied to a power MOSFET or BJT switch. The filtered power switch output voltage is fed back to a circuit that controls the power switch on and off times so that the output voltage remains constant regardless of input voltage or load current changes.
There are three common topologies: buck (step-down), boost (step-up) and buck-boost (step-up/stepdown). Other topologies include the flyback, SEPIC, Cuk, push-pull, forward, full-bridge, and half-bridge topologies.
Switching regulators require a means to vary their output voltage in response to input and output voltage changes. One approach is to use PWM that controls the input to the associated power switch, which controls its on and off time (duty cycle). In operation, the regulator's filtered output voltage is fed back to the PWM controller to control the duty cycle. If the filtered output tends to change, the feedback applied to the PWM controller varies the duty cycle to maintain a constant output voltage.
Boost converter
A boost converter (step-up converter) is a DC-to-DC power converter with an output voltage greater than its input voltage. Boost converters are used when it required higher voltage than available voltage from battery. Suppose you have a 3.7 V battery but you need 5 V for your device then you can use boost converter.
Because of the ease with which boost converters can supply large over voltages, they will almost always include some regulation to control the output voltage, and there are many I.Cs. manufactured for this purpose A typical example of an I.C. boost converter is the LM27313 from Texas Instruments. This chip is designed for use in low power systems such as PDAs, cameras, mobile phones, and GPS devices. Another common adjustable boost converter is LM2577.
Step 13: Integrated Circuits
An integrated circuit (IC), sometimes called a chip or microchip, is a semiconductor wafer on which thousands or millions of tiny resistors, capacitors, and transistors are fabricated. Integrated circuits (ICs) are a keystone of modern electronics. They are the heart and brains of most circuits. An IC can function as an amplifier, oscillator, timer, counter, computer memory, or microprocessor. A particular IC is categorized as either linear (analog) or digital, depending on its intended application.
Linear ICs have continuously variable output (theoretically capable of attaining an infinite number of states) that depends on the input signal level. As the term implies, the output signal level is a linear function of the input signal level. Ideally, when the instantaneous output is graphed against the instantaneous input, the plot appears as a straight line. Linear ICs are used as audio-frequency (AF) and radio-frequency (RF) amplifiers. The operational amplifier(op amp) is a common device in these applications.
Digital ICs operate at only a few defined levels or states, rather than over a continuous range of signal amplitudes. These devices are used in computers, computer networks, modems, and frequency counters. The fundamental building blocks of digital ICs are logic gates, which work with binary data, that is, signals that have only two different states, called low (logic 0) and high (logic 1).
Depending on the way they are manufactured, integrated circuits can be divided into two groups: hybrid and monolithic. Hybrid circuits have been around longer.
IC Packages
The package is what encapsulates the integrated circuit die and splays it out into a device we can more easily connect to. Each outer connection on the die is connected via a tiny piece of gold wire to a pad or pin on the package. Pins are the silver, extruding terminals on an IC, which go on to connect to other parts of a circuit. These are of utmost importance to us, because they’re what will go on to connect to the rest of the components and wires in a circuit.
There are many different types of packages, each of which has unique dimensions, mounting-types, and/or pin-counts.
Pin Numbering
All ICs are polarized,and every pin is unique in terms of both location and function. This means the package has to have some way to convey which pin is which. Most ICs will use either a notch or a dot to indicate which pin is the first pin. (Sometimes both, sometimes one or the other.)
Mounting Style
One of the main distinguishing package type characteristics is the way they mount to a circuit board. All packages fall into one of two mounting types: through-hole (PTH) or surface-mount (SMD or SMT). Through-hole packages are generally bigger, and much easier to work with. They’re designed to be stuck through one side of a board and soldered to the other side.
Surface-mount packages range in size from small to minuscule. They are all designed to sit on one side of a circuit board and be soldered to the surface.
Common ICs
Logic Gates (7400 series), Timers (555, 556), Shift Registers (74HC164, 74HC595), Microcontrollers (PIC16F877A, ATmega328P), Microprocessors (8086, 80386, MC68030), FPGAs, Sensors(LM35, 5843), RTC (DS3231, DS1307), Etc.
Step 14: References
Most of the image are taken from internet. Some idea and text are taken from:
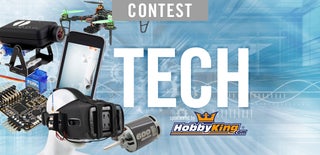
Runner Up in the
Tech Contest
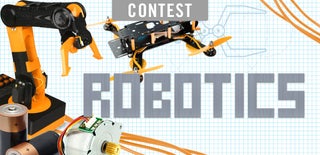
Participated in the
Robotics Contest