Introduction: Cosmos Mariner: a Large Aperture Dobsonian Telescope
There are many good Instructables here about making your own backyard Dobsonian telescope. Many of us start in the 8-inch to 10-inch range, and spend many long nights cruising around the Cosmos checking out the sights. Eventually, however, many of us are afflicted with a longing called "aperture fever" -- the desire to have a much larger telescope, that can gather just a few more bits of light from the Cosmos. This Instructable will outline my own response to aperture fever, the construction of a telescope that I call "COSMOS MARINER."
Ever since I started out in amateur astronomy, I have named my telescopes. It is a habit I learned from reading David Levy (my favorite book of his is "Guide to the Night Sky", which used to be called "The Sky: A User's Guide"). I always knew I was going to build a large telescope, and had waffled around with names. One of the formative experiences in my life that firmly put me on the path to being a professional scientist was watching Carl Sagan's Cosmos. Further, my youth was filled with incessant study of deep space missions, and I was well aware of the Mariner series of spacecraft, notably Mariner 9, the first spacecraft to visit Mars. Additionally, I had been impressed by Gale Christianson's book, "Edwin Hubble: Mariner of the Nebulae". Somewhere along the way, it all got put together to become Cosmos Mariner, or "Mariner" for short.
Before we dive into the project, I have to thank my wife. This project would not have been possible without her support, patience, and willingness to simply let me build the telescope I wanted to build. She never blinked an eye through the entire long process. She listened to me agonize and rant about how to do this that or the other thing, and nodded like she belived me everytime I promised that the views of the Universe would be more than little white smudges when it was finally done!
I've made many steps in this Instructable to make the supporting text more closely connected to the pictures I show. It makes the project look long, and it is! Constructing a telescope this big is no small endeavour; it took about a year to complete, working a couple of hours on my weekends each week.
Step 1: Amateur Telescope Making Resources
Amateur Telescope Making is a rewarding hobby, and the amateur astronomy community spawns many excellent innovations and designs. The design of Mariner is a classic design known as a "truss Dobsonian", with a few special modifications and choices made to reflect my own personal preferences and observing habits. This telescope is also HUGE, but it is completely scalable to a smaller (or bigger!) telescope of similar design. In fact, the original Mariner design is closely based on my smaller telescope I designed and built, named Equinox.
A scope the size of Mariner is probably not the best "first" project, but for those who are starting on the large telescope journey, hopefully this provides some ideas about how the process goes and things you may try. For those who dream of building a big scope, hopefully it will provide some idea of what you can expect when you start down this road.
By my count, this will be the ninth telescope I've built for myself, family or friends. Amateur Telescope Making combines woodworking and shop skills with a fair amount of design work, all of it necessary to insure your telescope works by the time you're done! Building a telescope is not something I did on my own; I had lots of help and inspiration from many sources.
If you are a first time telescope builder and want to try something smaller and more manageable for your first project, then I highly recommend "Build Your Own Telescope" by Richard Berry. It has the impressive subtitle "Five telescopes you can build with simple handtools." I've built several of the telescopes in this book, and he delivers on this promise. I also quite like "Making & Enjoying Telescopes: 6 Complete Projects & A Stargazers Guide" (by Robert Miller and Kenneth Wilson). Both are great books for starting out, and I still thumb through them for inspiration about the craft.
For big telescopes, however, one of the standard references is "The Dobsonian Telescope: A Practial Manual for Building Large Aperture Telescopes" (by David Kriege and Richard Berry). It has lots of specific details about building modern Dobsonians, some of which I follow, and some of which I don't.
This Instructable will take you through the entire process of building Mariner, which follows roughly the outline presented in "The Dobsonian Telescope."
Step 2: Vocabulary
If you're new to telescope building, some of the language we use can be a bit bewildering (or at least I remember that was the case). So here is handy guide to refer to so you can map what I'm saying onto the picture of the telescope.
- Bearing: A surface that the telescope turns on. There are two in a Dobsonian -- the bottom of the rocker box, and the two large circular side bearings.
- Diagonal (Secondary Mirror): This is a small flat mirror that is directly over the primary mirror, but suspended in the secondary cage. It takes the focused light from the primary mirror and redirects it out the side of the telescope into the eyepiece.
- Finder: A small device near the eyepiece that is used to point the telescope. A "finderscope" is a small, low power telescope, about as powerful as a pair of binoculars.
- Focuser: The focuser is your primary interface with the telescope. It holds the eyepiece, which you look through to see the telescope's view of the sky.
- Ground Board: The bottom piece of the rocker box that sits directly on the ground.
- Mirror Box: The lower square assembly that holds the primary mirror cell and mirror. The truss poles mount to the top side of the mirror box.
- Mirror Cell: A metal frame to securely hold the primary mirror and allow you to make finescale adjustments to align all the optics.
- Primary Mirror: In Newtonian telescopes, the mirror is what gathers all of the light. It is the most important (and expensive!) piece of the telescope.
- Rocker Box: The lowest main assembly. The entire telescope sits and pivots on this structure.
- Secondary Cage: The upper cylindrical assembly that holds the secondary mirror, the focuser, and the finderscopes. The truss poles mount to the bottom side of the cage.
- Shroud: A fabric cover that stretches over the truss poles to prevent stray light from making it into the telescope.
- Spider: The mechanical structure that holds the secondary mirror rigidly in place in the secondary cage.
- Telrad: A Telrad is a "unit finder" that operates like a heads-up display. To point the telescope, you look through a small pane of glass which has a luminous bulls-eye projected on it. There is an excellent Instructable about making your own.
- Truss Pole: One of the eight poles that provide the rigid body of the telescope, holding the secondary cage and mirror box together and in alignment.
I have an additional personal vocabulary I use to know which side of the telescope I'm talking about. The side with the eyepiece (the side you stand on when observing I call the "eye" side. The opposite side I call the "far" side. The scope pivots up and down on its bearings. The side that is below the telescope when it pivots down is called the "ground" side, and the side that is above is called the "sky" side. I mark pieces (like the truss poles and the side bearings) with these names to make assembly in the field easy and straight-forward.
Step 3: Sources of Parts
Building large aperture telescopes is not a cheap endeavour. Depending on how industrious you are, you can fabricate many of the bits and pieces yourself (especially if you have some experience with metal working). I'm mostly a wood-worker, so I did all the wood, and left machining and metal parts to others. The cost of building a telescope in the size range of Mariner is between $5,000 and $10,000, largely driven by the cost of the primary mirror.
The primary cost driver is the primary mirror -- as a general rule, it is advisable to spend as much money as you can on your mirror, since it is the one piece that is doing all the work. The entire project took about a year to construct (mostly done while I was waiting for my mirror to arrive), so that helps spread the cost out, but it does add up. The list below captures all the core components that I purchased and the vendors I used; you can visit their sites for current pricing and (often) other options:
- Primary Mirror -- Zambuto Optical
- Secondary Mirror -- Ostahowski Optics
- Secondary Spider -- Astrosystems
- Focuser -- Moonlite Telescope Accessories
- Truss Pole Clamps & Cage Clamps -- Aurora Precision
Step 4: Deciding on Optics
With any telescope building project, you begin with the optics. For big telescopes, it can take a year or more for your mirror to be fabricated (or a year or more to make your own, if you do that sort of thing), so you have to decide from the outset how big you want it to be. This is also usually the cost driver for the telescope.
When I go out observing, I like to look at galaxies. There is something deeply rewarding about hunting and pecking around the sky, navigating through vast fields of stars until eventually, you see a tiny smudge of light that is your quarry. They are often odd shapes and variously bright or dim as you look from the outside to the inside. But what amazes most, every single time, is knowing that the light had travelled millions and millions of light years to me at that moment. I love that.
Which means I very often find that I'm pushing my scopes to the boundaries of what they are capable of seeing, always looking for the faintest galaxy I can. That is usually when the aperture fever kicks in -- I know if I had a bigger telescope, then I could see fainter galaxies. When I think about increasing the aperture of my telescope, I always think of it in terms of "how muich fainter will I be able to see?"
Astronomers measure brightness with a number called the magnitude. The bigger the number, the fainter the object (it's weird if you aren't used to it; it's a consequence of the way the ancient Greeks originally defined brightness). A good rule of thumb for calculating the faintest magnitdue (the "limiting visual magnitude") you can see with your eye through a telescope is:
- mlim = 9.5 + 5*log(D)
where D is the diameter of your primary mirror in inches. Before I built Mariner, I had two other telescopes: an 8-inch (mlim ~ 14) and a 12.5-inch (mlim ~15). For Mariner I wanted to get into the mlim ~ 16 range, and so was looking at mirror diameters in the 20-24-inch range.
Step 5: Mirror Orders
Many amateur telescope makers enjoy making mirrors. Small mirrors (less than 12-inches) are not difficult to make by hand, and people can craft fine optics for their telescopes in their garage at home. Larger than 12-inches can also be made at home, though the process is more intensive and requires more skill, and possibly the construction of machines to help with the grinding process.
I've never enjoyed making optics, so I purchase my mirrors from master opticians who know the artform well, and then I build telscopes around the mirror. For large aperture telescopes (20-inch or larger), there are several well known mirror makers in North America, including Swayze Optical, Steve Kennedy, and Mike Lockwood. My mirror was made by Carl Zambuto at Zambuto Optical. I ordered a 22-inch diameter mirror, with a focal ratio of f/5 (a focal length of 22 x 5 = 110 inches). It took Carl about 9 months to make my mirror, and he was gracious enough to send me a few pictures of the process along the way.
A reflecting telescope is not just one mirror -- it is two mirrors. All the light gathered by the primary is reflected up to a small flat mirror (called the secondary) that reflects it out the side of the telescope to the focuser and the eyepiece. I got my secondary mirror from Terry Ostahowski at Ostahowski Optics.
Step 6: Design Plans
Once I had ordered my mirrors, I could start planning and building the telescope. The reality is that I designed and built each of the major components one piece at a time, but I present the designs here collected in one place for reference. I've always worked measurements and dimensions out by hand, and sketched things out in Illustrator, which is what I did for this project. However I think next time I do this, I should probably learn to use some modern CAD design software to help (for my next project, I'm going to try and use TinkerCad as a starting point).
The superstructure of the telescope has four major components: the mirror cell (described in the next step), the rocker box, the mirror box (and bearings), and the secondary cage. The plans above represent the final evolved thinking that went into the design, and have some of my notations about why I made the decisions I made and the materials I ultimately used on the scope. I didn't keep such detailed records when I made my 12.5-inch telescope, and wished I had, so for Mariner getting them down on paper was important (so I'll be ready to build my next big telescope!).
The Mariner design is a classic truss-Dobsonian design, but there are a few things that I insist on that deviate from what other amateurs might do. These include:
- You should always have a primary mirror cover, to prevent things from being dropped on the mirror when you aren't observing -- the mirror is the single largest cost item in the telescope, and should be protected. Many telescopes have a removable mirror cover, but then you have to find a place to store it at night where it doesn't get stepped on! I had designed an internal fold-up mirror cover on my 12.5-inch, and wanted a similar system on Mariner.
- I don't use a computer to navigate my telescope -- I'm a visual observer who "star-hops" using a start chart. To do this, you usually have some smaller navigational devices on your telescope to help get the telescope pointing in the right area of the sky. I have two: a Telrad and a finderscope. Some amateurs have one or the other, but I find them both useful in different situations. The Telrad is a "reflex sight." It has a diagonal pane of glass that projects a red bullseye on the sky like the heads-up display in a fighter jet. A finderscope is simply a small telescope that has a much wider field of view -- it is useful for navigating from small star to small star as you work your way across the sky from something you can easily find to your target.
- There is a general trend to make the secondary cage assembly as small as possible. I like to have my focuser near the back of the cage, and my Telrad and finder forward of this, so I can simply turn my head to use them without having to physically move my body. This is important, since as we will see, using this scope means observing on a ladder a lot. The net result is my secondary cage is a bit longer than on most scopes.
Step 7: Making Big Circles
At many steps throughout this process, the design calls for large, precise circles cut out of wood. If you spend time browsing the web, wood-workers have many tricks and techniques for this particular problem. Modern computer-controlled router systems like X-Carve can also cut them (though for Mariner, the bearing cuts are too large even for the largest 1000mm x 1000mm X-Carve).
For this project, I made all the large circular cuts using a center-pivot router jig, making multiple shallow passes with a straight router bit to cut all the way through a piece of wood. The base of my plunge router has two slots to accept rods from a jig of this sort. I made a jig by using two long pieces of threaded rod, securely mounted on a block of 2x2 lumber using bolts and washers. In the center of the block is a hole for a long decking screw, which I can drill down into the pice of wood I am cutting the circle out of.
To make a circular cut, you screw the wood block down at the center of the piece you want cut, and fix the router at the radius of the circle you want. Lower the router bit only a small bit, 1/16 to 1/8 inch, and make a cut along the full length of the arc you need. Extend the router bit down another 1/16 to 1/8 inch, and make another pass. Do this repeatedly until you have completely passed through the piece of wood. You'll need a "waste board" underneath your piece -- your pivot screw usually goes all the way through into this, and the last pass with the router bit goes all the way through your work piece.
This process is time consuming, but makes precise and clean circles, ideal for mechanical structures like telescopes! For smaller circles, I made a smaller jig out of a scrap of hardboard.
Step 8: Mirror Cell Planning
After the primary mirror itself, the mirror cell is probably the next most important piece of the telescope. It holds the mirror and keeps it from deforming, and it protects the mirror while the telescope is being transported. I don't have any experience machining metal, nor the tools in my shop to do it, so I designed my mirror cell and had a local machine shop make my cell.
The mirror cell has two basic parts: a frame that all the pieces are mounted to, and a floating set of points that the mirror sits on. The points are calculated to sit in precise locations to provide even support across the mirror. The support points are generally mounted on the points of triangles, which are themselves supported above the mirror cell on a whiffle-tree of metal bars that allow them to pivot and tilt together.
There are a variety of other pieces that also mount onto the frame to keep the mirror in place. These include the mounts for a sling that supports the lower edge of the mirror, side pins that keep the mirror from sliding back and forth, and leveling screws that connect to the whiffle-trees holding the mirror to provide adjustments to the optical alignment ("collimation").
To lay out my mirror cell, I started by evaluating where the support points needed to be. I used a bit of advice from Kriege & Berry, but calculated numbers using a finite element analysis program known as PLOP. PLOP takes the thickness of your mirror, the planned number of support points you are expecting to have, and then predicts the expected deformations in the surface of the mirror. It then automatically calculates the precise size of the triangles and bars for your mirror cell needed to minimize that distortion. For mirrors my size, the standard choices for supports are either 18 points of support, or 27 points of support. I simulated both, and decided the 18-point support would be good enough. The added complexity of the 27-point support trees would not pay off in the support gains on the mirror.
The rest of the cell was laid out using a lot of trigonometry and my calculator!
Since I was having a local machine shop do the cell for me, I had to provide two bits of information: the fabrication instructions for each piece that would go into the mirror cell, and then the assembly instructions for how to weld the cell together.
Step 9: Mirror Cell Fabrication
Amateur astronomers will argue about every aspect of building your own telescope, including what the appropriate materials are. I chose stainless steel for my mirror cell; since my machine shop was doing all the work, I didn't have to worry about the difficulty of working with the material itself. The shop did a great job, and were kind enough to take some pictures for me during the fabrication.
Once I got the cell home, there were many cycles of assembly and disassembly to test fit parts and prepare for building the rest of the scope around the cell. Inside the telescope, you want as many surfaces to be non-reflective as possible, to reduce stray light and enhance the contrast of your images, so I had the entire mirror cell powder-coated a flat black.
Step 10: Mirror Cell Collimation & Mirror Support
The 18-point support system is composed of three bars, with a triangle mounted on the end of each bar. The triangles are mounted at their centers with a pan head screw, secured from underneath with a metal lock-nut. The extra length of the screw goes through the end of the horizontal bar, and is terminated with another metal lock-nut. Thick felt furniture pads are placed on each point of the triangle for the mirror to rest on.
At three points in the frame of the mirror cell, appliance leveling feet are are inserted in threaded holes that were put in the frame bars. Each of these leveling feet is attached to one of the three bars holding the triangles using lock-nuts. The bars can pivot and move freely, but not drastically so. This construction holds all the six triangles in a plane for the mirror to rest on.
Twisting the leveling feet in or out one at a time changes the tip and tilt of the mirror ("collimation" in telescope speak) to align all the optics in the telescope. Once the triangle assemblies and collimation knobs are in place, a wire is threaded around the inner point of all six triangles, to keep them properly oriented toward the center of the mirror cell.
Step 11: Mirror Cell Hardware
At three points around the edge of the perimeter of the mirror outline, long carriage bolts stand up taller than the mirror. Each of these holds a delrin cylinder that is just taller than the mirror when it is sitting on the triangles, providing a bumper to protect the mirror from shifting around, particularly when you are transporting the telescope. The top of each of these bolts has an eye-bolt between lock-nuts that is covered with several layers of heat-shrink tubing (normally used to cover soldered splice joints in electronics). This construction is called a "mirror clip", and is rotated out to sit just above the mirror; these prevent the mirror from flopping forward out of its cell during transport (or during use, if your mirror was not properly balanced).
The last piece of mirror cell hardware is called the "mirror sling." The traditional sling is made out of a length of nylon strap, but in recent years a single steel cable system has become preferred since it allows your mirror to maintain a better overall shape. One of the most respected designs for cable slings was made by a member of the amateur astronomy community named Howie Glatter (so these are often called "Glatter slings"), but Howie sadly passed away in 2017. Currently Howie's classic slings are being made by the folks at Teeter Telescopes and can be purchased online. The bearings for the cable sling are mounted on vertical bolts away from the mirror edge, and the cable runs underneath the front edge of the mirror. It is kept on the mirror edge by being threaded through a small channel made of a couple of pieces of velcro, with a capture piece above the cable.
Step 12: Mirror Cell Fan
Large mirrors retain a lot of heat gathered during the day. That heat is slowly released at night and can have deleterious effects on views, particularly with larger mirrors. It has become common to have fans that circulate air onto the mirror to expedite the cooling time and break up layers of warm air that distort your views (this is often discussed as "boundary layer").
Many investigations have been done to decide what the best placement of cooling fans, with options being directly above, to the side, or directly behind. There is an additional choice about whether to have the fan push air, or pull air. I opted to put a single fan directly behind the mirror, pulling air.
I found the largest computer fan I could find, rated for the quietest operating sound to minimize the vibrations it put into the mirror frame. I ended up with a Silenx iXtrema Pro 120 fan, rated at 9 dBA for noise, and moving 38 cubic feet per minute.
I mounted it onto a small plate of 3mm hobby plywood, which is screwed directly into mounting points on the bottom of my mirror cell. There is a set of four sorbothane isolation washers between the wood mounting plate and the mirror cell, to reduce vibrations. The system is powered by an AstroSystems Cooling Fan System, which has two 7 amp-hour batteries. These batteries are mounted in the bottom of the mirror box (see Step 12) and contribute to the overall balance of the telescope.
Step 13: Mirror Box
The mirror box is one of the major structures in the telescope. The size is defined by the mirror cell, and by the desire to have the secondary cage nest inside the mirror box during transport. The lower corner on the ground side is cut diagonally off since inside the mirror box this is "dead space" outside the profile of the mirror and mirror-cell. By cutting the corner off, it lowers the pivot point of the telescope without it hitting the rocker box as it moves to its horizontal position.
The construction of the mirror box is simple: it is a box made with four pieces of 3/4-inch birch plywood, end-glued and screwed together. When the basic box is being fastened together, the squareness of the box is maintained using a set of corner clamps. As you can see in the picture above, the mirror box is large -- big enough to sit inside!
The interior of the mirror box has two major support structures. The first is a square piece of 1/2-inch plywood with a 24-inch diameter circle cut in it. This is called the "mirror baffle" and is mounted about 2 inches above the top piece of hardware in the mirror cell. The other support structure is a set of 4 "corner gussets" made out of 1/2-inch plywood, and mounted about 3-3/4 inches below the top edge of the mirror box. The mirror cell is bolted into the bottom of the box, which provides additional support to the structure.
The lower ends of the truss poles mount to the mirror box, just above the corner gussets. You can make wooden truss pole sockets, but I used a machined aluminum set from Aurora Precision (1.5-inch diameter truss poles).
Step 14: Mirror Cover
The mirror cover is built into the mirror box -- it is not a separate piece. It consists of four 6x26 inch pieces of 3mm hobby plywood. Two strips make one half of the cover, hinged at the seam between them to make a single folding door panel. The panel itself is attached to a thin 1x26 inch strip of 3mm hobby ply (to make the folding hinges level), and the strip is secured directly to the mirror baffle. When the mirror cover is deployed, the 4 panels lay side by side, flat on top of the mirror baffle, preventing anything from being able to fall directly on the mirror.
To uncover the mirror, the inner panels of each door fold over, backwards onto the outer panel, then the folded panel rotates up and away, and leans against the inner wall of the mirror box, out of the light path of the telescope.
Step 15: Laminating Wood for Rocker Box
The Rocker Box is the second major structure in the telescope, as is the sturdiest and heaviest piece, since it supports the entire telescope. Before building it you need to know the size of the mirror box, and also what your side bearing radius will be.
The rocker box has two components: the ground board, and the main rocker. The two are connected by a center pivot bolt, which provides one of the main motions of the telescope (rotating to point in any direction left or right).
The main rocker is heavy duty -- it has to support the entire structure of the telescope solidly without flexing. The bottom and sides are made of two laminated pieces of 3/4-inch birch plywood (for a 1.5-inch thickness). To laminate them together, I covered the entire area in wood glue, clamped completely around the edges, and stacked several hundred pounds of books in the center! It has been suggested you can apply weight to the center by parking your car up on top of the plate while it is bonding, but I didn't want to chance damaging the face of the wood.
Step 16: Rocker Box Base Bearing
The base of the rocker box is the easiest piece of the telescope to make -- it is perfectly square! Not to make it too easy, it has a hole drilled in the exact center for the pivot bolt. The bottom of the rocker box is one of the main bearings for the telescope -- a surface the telescope rotates on (the "azimuth bearing" in astronomer speak). For this bearing surface, I covered the entire bottom of the rocker box with a piece of Formica. Formica comes in a variety of different surface finishes. Amateur astronomers have discovered that bumpy surfaces are better than perfectly smooth surfaces. I used a piece of Formica 909-42 Sparkle Finish (the "42" type is the sparkle finish that has the bumpy surface that works so well).
I cut a piece of Formica laminate slightly larger than the base, and contact cemented it onto the bottom, using a roller to ensure good contact and adhesion across the entire surface. I used a laminate trim bit on my router to clear the excess edge off, then used a round-over bit to put a bevel completely around the outer edge to help keep it from lifting up.
Step 17: Rocker Box Sides
The sides of the rocker box mesh with the side bearings, so have to be cut to the same curve. I set up my router jig to make a large, constant radius curve (see Step 7). I didn't want to have to cut down through a full thickness piece, so I cut each layer of the side boards separately, then bonded the pieces together to make the full width side. Small ridges at the interface I cleaned off with my sander after the glue dried to make a smooth edge.
Step 18: Rocker Box Assembly
To secure the sides to the base, I used large lag bolts screwed up from the bottom of the baseboard into the rocker sides. This was done after the Formica was laid down. The lag-bolt heads are countersunk into the baseboard and left exposed, in case I ever have cause to deconstruct the rocker box.
The front and rear sides of the rocker box are single thickness panels of 3/4-inch plywood, glued and screwed into the thick sides.
Step 19: Rocker Box Ground Board
The ground board is mounted through the center of the rocker box base. It is made from a single thickness of 3/4-inch plywood cut in a triangular shape. It has a pivot hole directly in the center with a teflon pad, and three teflon pads out at each point of the triangle. The teflon interfaces with the Formica on the bottom of the rocker box to provide a smooth surface for the telescope to turn on. The ground board is sized so the outer teflon pads touch Formica just inside of the line of bolts used to mount the sides of the rocker to the base.
To keep the groundboard up off the ground and protect it against wear and tear and provide clearance for the pivot bolt, I made feet out of two pieces of plywood laminated together (cutouts from my side bearings, in Step **). I attach to these metal angle plates used to attach legs to furniture. The metal plate provides a hard interface with the ground that can easily be replaced if it shows too much wear and tear.
On the side of the ground board that faces the Formica on the rocker base, a 1.5x1.5 inch pad of teflon is screwed into the ground board, so the pads sit directly above the metal feet.
Step 20: Secondary Cage Rings & Struts
The secondary cage has to allow light to pass through to the primary mirror, but support all the hardware on the upper end of the telescope. The main structure is defined by two rings, each 1-7/8 inches wide, routered out of 3/4-inch plywood. The inner diameter is 24 inches. I used my router jig to cut full circles, first the outer edge, then the inner edge. To keep the piece secure when the final pass with the router cut through, I had screwed the ring down onto my wasteboard, by carefully locating the drill hole so they could be repurposed to mount the vertical struts.
The vertical struts of the secondary cage are laminations of two pieces of 3/4-inch plywood, with final dimensions of 1.5" x 1.5". The struts hold the two rings together, and provide the mounting points for the spider that holds the secondary mirror. The are oriented with the plys of the wood pointing radially inward to the center, so the spider vanes pull parallel to the plys, providing the most strength against bending. My spider was made by Astrosystems.
Step 21: Secondary Cage Hardware Boards
The secondary cage carries most of the hardware that interfaces you with the telescope -- your finderscopes and the focuser. Mariner has two boards that this equipment mounts to, each made out of a piece of 5/8-inch plywood. I have four pieces of hardware to mount:
- Focuser (from Moonlight -- a low-profile Crayford style focuser)
- A Telrad (a "unit finder" for pointing the telescope)
- An 80m Finderscope (for pointing the telescope)
- A green laser pointer mount (also for pointing the telescope)
The boards screw directly into the rings on top and bottom. I laid it all out ahead of time to make sure I had all the pieces where I wanted them. The only major construction here is getting the mounting holes in the right places, and drilling a 2-1/4-inch diameter hole for the focuser tube to descend into. This hole has to be placed in a location where it will look at the secondary mirror once it is mounted on the spider, which means it has to be near the lower end of the board.
Step 22: Secondary Cage Liner
The secondary cage is usually lined with a lightweight material to block any stray light from reaching the eyepiece. The traditional material is Kydex plastic, but it is prone to damage and warps in the heat. I have always lined my secondary cages with a piece of Formica. It is very tough and durable and does not add a dramatic amount of weight. I used a piece of black Formica 909-58 Matte Finish cut to size. It has to be bent slowly so it doesn't crack, and is secured to the cage rings with small wood screws. The seam is placed directly behind the focuser board; I had to cut a little notch for the focuser. I also line the inside of the Formica with flocking paper, a flat black velvety material, to reduce stray light. You could also spray paint the inside of the Formica flat black.
Step 23: Side Bearings
To my mind, the side bearings were the hardest part of the project. They are all curves, which means there was a lot of router work to cut them out. They are 1-5/8 inch thick, made of three layers of birch plywood: 1/2-inch + 5/8-inch + 1/2-inch. The width was chosen to match the sides of the rocker box exactly, then hold the mirror box away from the sides of the rocker box with a 1/8-inch gap on both sides. As I did with the rocker box, I made each piece separately then glued them together.
A good rule of thumb is to have bearings roughly the size of your mirror. The smaller the bearing, the harder it is to pivot your telescope. I chose to make LARGE bearings, with a diameter of 43-3/4 inches. The reason for this was I wanted the pivot point to be high up on the scope (to help balance the structure), but keep the rocker box short. I also think it looks cool to have the big side bearings. :-)
The outer edge is the main surface that forms the bearing for the telescope to pivot up and down (the "altitude bearing" in astronomer speak). The inner curved edge is for aesthetics mostly, but also removes excess weight -- several layers of plywood bonded together are very heavy! There are three holes drilled out of the center of each bearing, again for aesthetics. This was a design element I had used on my smaller scope, and repeating the three hole pattern here points to the heritage of both scopes together.
To make the bearing surface, I contact cemented a long thin strip of the Formica 909-42 Sparkle Finish (same thing used on the bottom of the rocker box) on the outside rim of both bearings. A wood screw through a finishing washer is located at each end, to prevent the Formica from lifting up.
Step 24: Truss Pole Length Determination
In principle, you should be able to compute how long the truss poles need to be. They should be an appropriate length so the mirror brings light to the secondary mirror, which then puts the light at the right location for an eyepiece to focus and make a clear image. Over the many telescopes I've made in my days, I've found the most effective and reliable thing to do is simply set up the scope and measure how long the truss poles need to be!
So I set it up in my driveway one afternoon on a rough jig to hold the mirror box and cage aligned and moved them back and forth until all of my eyepieces focused on the distant landscape. This defines the length of the truss poles so I could cut them to correct length.
Step 25: Truss Pole Mounts
The truss poles are covered in black pipe insulation foam, to keep them from condensing water in the cool evening air, and also to cover up the shiny silver surface. I used pipe foam for 1-5/8" pipe to cover my 1.5-inch diameter trusses -- I cut a small strip off so it fit snug, and secured a piece around each truss pole with velcro ties.
The truss poles are mounted using hardware from Aurora Precision. At the bottom are truss pole clamps mounted to the mirror box. These are open slots for the poles to slide down into, and then the clamp secures them.
The top is more complicated. Two truss poles are joined together by a bracket that pivots back and forth. The bracket is permanently attached to the truss poles with a bolt that mounts in a star type threaded insert that has been pounded down into the truss pole. The bracket mates with cage clamps that are permanently mounted on the bottom of the secondary cage.
Where you choose to mount the cage clamps determines where the eyepiece will be. The conventional choice (which I used) is to have the eyepiece come directly out the side, but some people like to have the eyepiece rotated 45 degrees toward the SKY side of the scope, so when it is pointing near the horizon the eyepiece is easier to get to. This is a good choice for small telescopes, but on large ones like Mariner if they eyepiece is rotated in this fashion you would have to lean over the scope while on a ladder to reach it.
Step 26: Balance & Bearing Mounts
Once the truss poles were the correct length, I could find the telescope's balance point. The balance point is the line through the telescope where I want the center of the bearings to sit, so it stays as well balanced as it can no matter how far up or down it is pointing.
To find the balance point, I put the entire scope together, then laid it across a large (2-inch diameter) dowel. I then rolled the scope back and forth on the dowel until it balances perfectly and doesn't tip forward or backward. Noting that point on the scope, I then determined where the bearings would have to sit for their center (virtual center -- here "center" means the center of the circle that matches the outer arc of the bearings) to be on the same line. With that in mind, I marked on the back of the bearing where it overlaps with the mirror box, so I could lay out where to put the mounting bolts that secure the bearings to the mirror box.
The bearings are designed to be removable, so the scope can be transported in a smaller space, and the mirror box can be handled without the weight of the bearings if it needs to be. They are mounted to the mirror box using 1/4-inch socket cap screws that pass through the bearing, through the side of the mirror box, and thread into tee-nuts on the inside of the mirror box. There are seven on each bearing. I worked out the locations post-facto after I knew where the bearing was going to overlap the mirror box.
Step 27: Rocker Box Rider
Every big project has glitches that aren't discovered until you are deep into the building. For Mariner that glitch had to do with the side bearings. They are so large that when the telescope is pointed near horizontal, the mirror box is completely clear of the rocker. At this point the telescope is riding on the side bearings, but a little push will knock it off the bearings and onto the ground!
The solution was to build small riders out of 3mm hobby plywood. These are restraining rails that sit at the bottom of the rocker box arc, and always provide a surface that constrains horizontal shifting of the telescope. They were constructed from two pieces glued together and screwed into the rocker box. There is a piece of felt lining to make sure they run smoothly against the side bearing as it is turning.
I cut three holes into each one to match the motif of the side-bearing, so it kind of looks like I planned it this way from the beginning. :-)
Step 28: Shroud
The beauty of the truss Dobsonian design is it is airy and light (relatively speaking -- Mariner is pretty heavy!), but it is still desirable to keep light out of the main truss, lest it finds a way to make it to the mirror and reduce the contrast of what you are looking at.
The solution is to make a shroud of fabric that can go around the truss poles. Spandex is a traditional material that gets used, but it has the interesting property of getting tight when its hot (that's why it looks good as exercise clothes!), and expanding when it gets cold (so it sags on your scope at night!). Instead, I use stretchy T-shirt material, 96% cotton/4% spandex.
The shroud is a simple affair -- a long slightly tapered tube with only one seam. The overall lengths around the tube ends are the perimeter of the mirror box and secondary cage respectively. The ends are sewed over so a drawstring can go through them, and the drawstrings are cinched at the cage and at the mirror box. For the strings I used some reflective camp cord with spring loaded cord locks for draw strings.
Mariner's shroud is huge, so I needed a way to tell the top from the bottom, especially in the dark. So I sewed a small patch on one end. Just for orientation purposes. :-)
Step 29: Finishing
There is a strong tradition in the home-built truss Dobsonian community to have the scope be "natural wood" tones, but I like a splash of color. I matched the scheme on Mariner to the one I used on my previous scope, staining the mirror box and the focuser boards a transparent green so the grain shows through, and leaving the other pieces natural.
Once the stain was laid down, I coated everything in marine grade spar urethane. The inside of the mirror box got one coat of urethane to seal it, then it was painted flat black. All other surfaces received three (3) coats of spar urethane, sanding lightly with 220 grit sand paper between coats. This gives the outside of the scope a durable, glossy finish that can hold up to the cold and the dew when out observing at night.
I had an engraving company make a plaque for me out of anodized aluminum. It sits right over my eyepiece, and has a quote from Vincent van Gogh, written in a letter to his brother Theo while he was resident at Saint Remy. It says : "I know nothing with any certainty, but the sight of the stars makes me dream."
Step 30: Handles & Wheelie Bars
Mariner is a big scope, so moving it around is an engineering project in and of itself. The classic way to make large Dobsonians mobile is to construct detachable wheelie bars. These attach to the rocker box through tee-nuts on the inside, but let you tip the scope forward onto wheels and push it out into the backyard. This can be done easily if the scope is collapsed in its travel configuration, but can also be done over short distances with it assembled.
On the mirror box, I put two sets of handles on the GND and SKY side, so two people can lift just the mirror box if they need to. I also put two sets of handles on the front and back of the rocker box; you wouldn't want to lift the entire assembly with them, but you can lift the rocker box alone with them.
My wheelie bars were constructed by first bonding two six foot lengths of 1x4 hardwood boards together. On the "wheel" end, each bar has two 8-inch pneumatic tires. The tires use a long hex bolt as an axle, with a nylon lock nut to secure them in place.
The wheelie bar is attached to the rocker box using a captured eye-bolt that is bored through the wheelie bar and secured loosely with a nylon lock nut so it can turn freely; the lock nut is recessed into the bar so the wood of the bar is what makes contact with the telescope. The round side of the eyebolt is on the outside of the wheelie bar and bears down on a large fender washer when it is tightened.
Two handles for lifting are placed on the inside ends of the wheelie bar on the opposite end from the wheels.
Each side of the rocker box has two holes bored through the side, with a tee nut on the inside to receive the wheelie bar eye-bolts. When the bar is attached, the wheel is about two inches off the ground, but when you lift up on the handle end, the scope tips forward onto the wheels and can be pushed around like a wheelbarrow.
Step 31: First Light!
In a big project, you eventually reach that point where it is done. With telescopes, the first night out under the stars is always special -- we call it "First Light."
We always starts early in the day, because we're eager to see some cosmic sights. We set Mariner up in the afternoon, when there was still light to see by. I rolled the scope out into the backyard, and took the wheelie bars off. It took a hour or so to get all the finderscopes lined up looking at landmarks on distant mountains. I checked and rechecked the collimation. We put the shroud on. And we waited.
The first thing we looked at (the first thing I always look at) was a double star in Cygnus called Albireo -- one of the stars is golden yellow, and the other is blue. We then proceeded to spend 6 hours touring around the sky, looking at stars and nebulae and galaxies and clusters. All in all, it was a fantastic evening, and I have enjoyed many since.
For all you amateur astronomers out there, our observed targets for that night were: Albireo, Mizar/Alcor, Polaris, M57, M13, 6207, M31/32/110, M103, 457, M11, Veil Nebula E/W, M51/5195, M27, Double Cluster, M8, M20, M17, M16, M15, M101, 7457, 7331, Stephan's Quintet, 891, 5907, 7662, TX Piscis (carbon), M92, M71, 7293, 7606, 7723, 7727, 7619/7626, 7448, M33, M45, Jupiter.
Step 32: Beauty Shots
Telescopes are definitely engineering marvels, machines built with elegant craftsmanship and creative problem solving. But if you're a telescope builder or user, they also have their own kind of beauty and artisianship about them. It's strangely appropriate that the machines we use to ponder the Cosmos are themselves awesome in form and function.
So I'll close this Instructable with some pretty pictures, and hope you are inspired in your own telescope-making journey! Good luck, and let us know how your own telescope journey is shaping up in the comments!
Step 33: Through the Scope [new!]
Several people in the comments have asked for images taken through the telescope. The views are awesome, but I don't do photography through Mariner. Even with a scope this large, photography of most things requires long exposures.That would require some tracking (an equatorial table should do the trick; there is a nice instructable on building one) and a camera and computer.
That being said, you can take pictures just holding your phone up to the eyepiece. This works pretty well with the Moon, and sometimes works okay with planets. All I can dig up on my drive are a few photos I snapped of the Moon. Pretty much any telescope will show you views of the Moon this well, but if you've never looked through a telescope it will give you some idea of what to expect.
Thanks for all the positive feedback and interest!
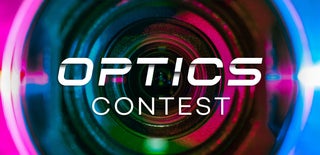
Grand Prize in the
Optics Contest