Introduction: Veggie Hydroponic Grow Box for Micro Gravity
Objective: Within a cube of 50cm dimensions grow 28 Lactuca sativa outredegous (Red Romain lettuce) plants hydroponically in a microgravity environment from seed to harvest without human intervention.
Further: Achieve maximum nutritional density in the shortest amount of time with minimal resources.
Supplies
The cube is constructed of a frame of:
12 Aluminum 7mm x 50mm L angles fastened together with
16 #6-32 x 3/8" machine screws and nuts. The side panels are
3 48.3 cm square corrugated plastic with diamond mylar covering the inner surfaces.
2 top and bottom panels are also 48.3 cm square corrugated panels with
6 45 cm x 1 cm 12V LED light strips glued to each panel. The light strips (waterproof) are a 3:1 red/blue with a combined power draw of 5 amps. They are powered by a Triac dimmable LED driver and are controlled with a dimmer switch.
1 80 mm x 80 mm 12V .2 amp fan is positioned in the top panel and connected to the same power supply and dimmer switch for the construction convenience of this otherwise static model.
Step 1:
1. Nutrient/Water delivery system to roots.
A hydroponic system will allow germination and growth without a soil growth media and avoid the necessity of cleaning the system between harvests. It is assumed for this model that cabin air temperature, humidity and CO2 levels are adequate for plant growth. Plant hydration and nutrition can be automatically regulated through the root system containment chambers which will be constructed so as to prevent water escaping into the cabin environment.
Step 2:
The foam seed containers placed into the root containment chamber openings will prevent water droplets from escaping into the cabin environment.
Step 3:
The liquid nutrients and air will be pulled through the root containment chambers by a liquid/air separator fan, similar in design to the ISS toilet system. This should create a negative pressure were the foam inserts are placed to further reduce the possibility of water droplet escape. The water and liquid nutrients initially flow from an inline two-liter reservoir. The reservoir also has an intake filter fed from cabin air to equalize pressure in the system as it exhausts air back into the cabin after exiting the liquid/air separator.
Step 4:
1.5” PVC pipe is used as the root containment chamber. 5/8” holes are drilled into the pipe 11 cm apart on both the upper and lower surfaces, 180 degrees opposite each other on the cylindrical surface. The chambers which are positioned horizontally in the middle of the cube structure will allow the plants to grow toward the light sources that are positioned inside the top and the bottom of the cube structure. A 19mm diameter by 25mm high polyfoam plug is placed in each hole. The plugs are slit down one side from the center of the cylinder. These are used to hold the seeds.
Step 5:
2. Energy Transfer
Advanced Plant Habitat (APH) light (Already in use by NASA) can be built into the top and bottom of the cube.This provides the ability to modulate the intensity and duration of the red, blue and green wave lengths as well as the full spectrum which can be programed to maximize energy transfer to the plant and consume minimum power. Due to the confined space the LED lighting will be placed in a position that would allow the mature lettuce outer margins to grow into contact with the light source. The challenge will be to keep the light from scorching the lettuce. To achieve this the LED’s should have an intensity control and probably a lens filter on the LED’s that can be manipulated so as to control the photosynthetic photon flux density (PPFD) to an optimal level. The PPFD should probably be reduced on a logarithmic scale based on the distance from the leaf tip to the light sources. The emission angle of incidence may also need to be taken into consideration. Once these controls are achieved this light source could also be used for different crops. The majority of the radiation will be in the 460nm and 650nm wave lengths.
Assuming that lettuce needs 17 hours of light per 24 hour cycle the daily light integral (DLI) should be DLI (mol m-2d-1) =0.0036 * PPFD (μmol m-2s-1) *17
Theoretical example: A focused hard stream of water can physically damage the cellular structure of a plant. If that intensity is reduced to a mist the water emission source can touch the plant without damaging the cellular structure of the plant. This effect is achieved by reducing the mass of the water droplet and the resulting force on the cell structure of the plant. While the mass or velocity of the photon cannot be reduced, is it possible to modulate the photons with some quantum filter in a manner so as to maintain the nutritional interaction with out the cellular degradation? Is it possible to decrease the photon emissions from the light source so as to maintain a relatively stable PAR (photosynthetic active radiation) value as the distance from the light source to the leaf surface decreases as the plant grows?
Step 6:
3. Air circulation
One or two fans need to be positioned in the cube to maintain constant cabin air flow to allow the leaves to breath in micro gravity. This will also allow the air intake for the reservoir and the exhaust air to be contained within the cube.
Step 7:
Current Model
Root interaction with a fluid and air mixture in microgravity can only be tested properly in microgravity. For this reason, I built a static non-working model to begin with. In order to partially validate the concept, I did make observations performing the following tests.
Step 8:
Germination
21 Lactuca sativa seeds were measured. Length variation 6.5mm to 8.5mm, Width 1.9mm to 2.6mm, Thickness 1mm to 1.3mm. These measurements were taken for consideration as to what size seed casing could be integrated in the root chamber.
11/25/19 I selected 5 Lactuca sativa seeds and placed them in a petri dish on a moistened fiber. The lighting was natural room lighting with several windows. The dish received direct afternoon sun through a glass door.
11/27/19 Cotyledons had emerged from four of the five seeds. From this observation it appears that the seeds should be placed in the seed container with the narrower end toward the direction that the plant should emerge
Step 9:
Seed casings
Cut 2.5 cm x 4 cm White polyester fiber 3 mm thick Cut 2.5 cm x 4 cm grey knit eyelet fabric 4 mm thick Placed some rock wool mineral fiber (Gro-dan) that had ben rinsed in 5.5 ph distilled water and rolled a small amount of the rock wool in both the grey and white materials and glued them shut with Super Lace wig glue (Isopropanol Ethyl Acetate Heptone).
Made 5 casings each with the grey knit and white polyester material. Made an indentation about 1 cm deep in the top of each seed casing and placed one seed (fatter portion of the seed) down into the rock wool.
Step 10:
In order to see how the seed casings worked a 1-1/4” clear vinyl tube with 3/16” thick wall was used. Ten 5/8” holes were drilled in a row with approximately 2” spacings.
The seed casings were placed into the holes with approximately 1 cm exposed above the outer wall of the tube. Ten drip lines were positioned at the bottom, of the horizontally positioned tube, one under each seed casing. The drip lines were connected to an irrigation timer. The period and duration were set so as to keep the seed casings moist during the germination period. The initial cycle ran for 30 seconds which filled the tube which was plugged at both ends. The leakage allowed the tube to drain in about 20 minutes. 12/12/19 First irrigation. Following irrigations occurred every 7 hours for a duration of one minute. The irrigation tank was initially filled with 8 liters of 5.7 ph water. The plugs at the ends of the tube were removed so the water now drains rapidly.
Step 11:
12/16/19 The first two cotyledons emerged. One that was wrapped with the white polyester and one wrapped with the grey knit. The ten specimens were then placed 20 cm beneath 2:1 red/blue LED grow lights 28-AC90 LED’s. The lights are left on for 17 hours continuous out of every 24 hours cycle.
Step 12:
12/17/19 After germination the following nutrients were added to the water:
10 ml Aqua A 4-0-1 10 ml Aqua B 1-4-2 The solution was re-stabilized to 5.8 ph 12/18/19 Added: 10 ml B+ 2-1-4 12/24/19
Only seed #10 in the grey knit has started to grow leaves. I removed the first 9 and examined the seeds. All seed casings had remained moist for the previous days. Seed #4 cotyledon had emerged so I left it in to observe further.
Step 13:
I replaced seed 1,2,3, and 5 and placed them in a foam seed holder 25 mm high by 19 mm diameter with a slit down one side from the center of the cylinder to the outer edge (commercially obtained). I did the same with seeds 6,7,8,9 but placed a small amount of rock wool next to the seeds. I wet the seed holders with the irrigation system till moist (45 second duration). I reduced the irrigation cycle to every 8 hours for 45 seconds. I added 28 ounces of 5.7 ph water to the reservoir to replenish what had evaporated.
Step 14:
12/28/19 Seeds 6,7,8,and 9 with the rock wool have begun to germinate. Seed #10 has roots extending to the bottom of the chamber. A blackout fabric was placed over a portion of the clear tube to protect the roots from the direct light. Seeds 1,2,3, and 5 have not germinated. This suggests the rock wool is needed to retain the proper moisture for germination.
Step 15:
1/4/20 (Unattended since 12/28/19) Seeds 6-10 have all continued to grow. It appears that some algae is growing around the base of the stems in the rock wool. Photo from 1/20/20
Step 16:
1/27/20 Harvested the plants from the 1-1/4” diameter tube. It appears the roots had more than enough room to grow suggesting a narrower diameter root chamber may be possible.
Step 17:
Even though some roots became entangled with each other, slowly pulling up on the foam plugs removed all the roots from the tube with a couple of small exceptions where roots had wrapped around the irrigation outlet at the bottom of the tube. This suggests that a smooth interior is desirable so that no cleaning of the chamber is needed between harvests. The water in this reservoir was never agitated so there was some settling of a small amount of brown particulate matter from the liquid nutrients over the 42 days. This suggest that the system may need to be flushed after some extended period of use.
Only plant #10 was in place for 42 days. Plants 6-9 were harvested after 34 days and could have been harvested sooner.
Step 18:
Conclusion
Having proved that the seeds can germinate in place and grow to maturity in the seed casings, success of the objective of growing from seed to harvest without human intervention is likely to be realized.
It still needs to be verified that the roots can uptake the nutrients in microgravity with the hydroponic flow, also should the flow be continuous or periodic.
The photonic energy transfer to the leaves has largely been worked out through existing systems which can be incorporated into this model through the additional experimentation with plant spacing and harvest intervals.
Oxygen, carbon dioxide, and plant transpiration can be adequately handled with fan circulation in the cube and into and out of the cabin.
The foam seed casings can be prepared on the ground with the seed and rock wool and packaged for storing. The seeds will germinate when placed in the root containment chamber once irrigation begins.
Replenishing the reservoir with water and liquid nutrients is the only maintenance required of the system on a regular basis. After replanting an Optical Mass Gauging System for Measuring Liquid Levels in a Reduced Gravity Environment will need to be temporarily plugged into the system to determine the amount of water and nutrients that need to be added to bring the system back up to the initial level.
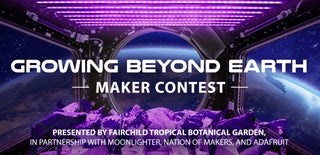
First Prize in the
Growing Beyond Earth Maker Contest