Introduction: Cloud Chamber: a Project to Detect Muons
Background
Fourteen billion years ago, when the hot dense speck, which was our universe, quickly expanded, all of the matter and antimatter that existed should have annihilated and left us nothing but energy. And yet, a small amount of matter survived. We ended up with a world filled with particles. And not just any particles – particles whose masses and charges were just precise enough to allow human life.
Search for the fundamental particles, the building blocks of all the matter, led to the discovery of molecules, atoms, protons, neutrons, electrons, mesons, pions, muons, neutrinos, quarks, bosons and so on. The search still continues… Important steps forward in the search of fundamental particles include the development of novel particle detectors. These are devices used to detect, track, and/or identify high-energy particles, such as those produced by nuclear decay, cosmic radiation, or reactions in a particle accelerator. The history of particle detectors dates back to more than 100 years ago. Photographic films and scintillation screens helped in the early days of nuclear physics to uncover the mysteries of radioactivity. Today, the scale of the detectors at the Large Hadron Collider is almost incomprehensible. They weigh thousands of tons, contain millions of detecting elements. However, from the 1920s to the 1950s, until the advent of the bubble chamber, Cloud Chambers played a prominent role in the experimental particle physics. In particular, the discoveries of the positron in 1932, the muon in 1936, both by Carl Anderson (who was awarded a Nobel Prize in Physics in 1936), and the kaon in 1947 (discovered by George Rochester and Clifford Charles Butler) were made using Cloud Chambers as particle detectors. Using the Cloud Chambers, Carl Anderson had detected the positron and muon in cosmic rays. Hence, I decided to make a Cloud Chamber and use it to detect the muons coming to earth from cosmic rays.
Step 1: Working Principle of Cloud Chamber
A simple cloud chamber consists of a sealed chamber containing a supersaturated vapor which is on the point of condensation. Highly pure (more than 90%) Isopropyl alcohol which allows easy evaporation is used as a source of vapor. A cold metal plate, being kept cooled using dry ice is kept at the bottom of the chamber. As the alcohol vapor falls and comes into contact with the cold metal plate, it cools down. Just above the cold metal plate, there is an area of the chamber where most of the alcohol has not condensed, and the result is a supersaturated vapor which is on the verge of condensation. When a charged particle (for example, an alpha or beta particle or muon) passes through this supersaturated vapor, it ionizes the vapor molecules with which it interacts. The resulting ions act as condensation nuclei, around which surrounding vapor molecules condense and a mist is formed, because the vapor is on the point of condensation. A high energy charged particle passing through the supersaturated alcohol vapor produces many ions along its path and leaves behind a trail of mist.
Using the above principles, a Cloud Chamber is used to detect ionizing particles and to determine their trajectories. It does not show the particles themselves, but where they have been. Particles form a condensation trail in the chamber which is visible by naked eye as a fine mist, and this shows a particle's path through the chamber. They can include any electrically charged particle that passes through the chamber, and the amount of ionization can be deduced from the tracks in the chamber and is used to determine a particle's identity. Cloud chambers are particularly used to study radioactive elements, as alpha, beta and gamma radiation are all ionizing (with alpha being the most so). Cosmic rays can also be detected by cloud chambers, as the secondary rays formed in particle showers are ionizing particles such as muons and electrons. When a cosmic ray zips through a cloud, it creates ghostly particle tracks that are visible to the naked eye.
Step 2: COSMIC RAYS AND MUONS
Cosmic rays are immensely high-energy radiation, mainly originating outside the Solar System. They are composed primarily of high-energy protons and atomic nuclei. When cosmic rays enter the Earth's atmosphere they collide with atoms and molecules, mainly oxygen and nitrogen. Typical particles produced in such collisions are neutrons and charged mesons such as positive or negative pions and kaons. Some of these subsequently decay into muons, which are able to reach the surface of the Earth, and even penetrate for some distance into shallow mines.
The muon is an elementary particle similar to the electron with an electric charge of -1 e and a spin of ½, but with a much greater mass. The muon is an unstable subatomic particle with a mean lifetime of 2.2 microsecond. Among all known unstable subatomic particles, only the neutron has a longer decay lifetime (about 15 minutes). Other decay significantly faster. Muons have a mass of 105.7MeV/c2, which is about 207 times that of the electron.
HOW MUONS ARE ABLE TO REACH THE SURFACE OF THE EARTH
When a cosmic ray proton impacts atomic nuclei in the upper atmosphere of the Earth, pions are created. These pions decay within a relatively short distance (meters) into muons (their preferred decay product), and muon neutrinos. The muons from these high energy cosmic rays generally continue in about the same direction as the original proton, at a velocity near the speed of light (0.98 times the speed of light). However, they have a mean life-time of only about 2.2 microseconds. Therefore, without relativistic effects, their lifetime would allow a half-survival distance of only about 448 m (2.2 µs×ln(2) × 0.98×c) at most. However, from the viewpoint of the Earth, the time dilation effect of special relativity allows cosmic ray secondary muons to survive the flight to the Earth's surface, since in the Earth frame, the muons have a longer half-life due to their velocity. From the viewpoint (inertial frame) of the muon, on the other hand, it is the length contraction effect of special relativity which allows this penetration. Both effects are equally valid ways of explaining the fast muon's unusual survival over large distances. According to the time-dilation, time dilates for any object that is moving with a velocity. This dilated time can be calculated using the Lorentz factor: T = To/((1-u^2/c^2)^0.5) where,
T is the dilated time
To is the rest half life
u is the speed of the particle
c is the speed of light and
'^' indicates 'to the power'
From the equation, it can be implied that higher the speed of the particle, higher is the time dilation. For a muon, the rest half life is 2.2 x 10-6 sec and velocity is 0.98 times the speed of light (0.98 c). Therefore the dilated time comes to 11 x 10-6 sec. Due to this, the range of the muon increases significantly.
Had there been no time dilation, the range of the muons would have been so small that they would not have been able to reach the surface of the Earth. But since we are able to observe their trails in the cloud chamber, this verifies Einstein’s theory of time dilation. Thus, apart from detecting the sub-atomic particles, the cloud chamber is also helpful in verifying the Einstein's Theory of time dilation and length contraction.
Step 3: Identification of Particles Based on Their Tracks
Many different types of particles pass through the cloud chamber. Though they are difficult to be seen, but they can actually be identified based on the tracks they leave behind, as explained below:
Short, fat tracks: When we see short, fat tracks, we’re seeing an atmospheric radon atom spitting out an alpha particle (a clump of two protons and two neutrons). Radon is a naturally occurring radioactive element, but it exists in such low concentrations in the air that it is less radioactive than peanut butter. Alpha particles spat out of radon atoms are bulky and low-energy, so they leave short, fat tracks.
Long, straight track: These are muons! Muons are the heavier cousins of the electron and are produced when a cosmic ray bumps into an atmospheric molecule high up in the atmosphere. Because they are so massive, muons bludgeon their way through the air and leave clean, straight tracks.
Zig-zags and curly-cues: These are either an electron or positron (the electron’s anti-matter twin). Electrons and positrons are created when a cosmic ray crashes into atmospheric molecules. Electrons and positrons are light particles and bounce around when they hit air molecules, leaving zig-zags and curly-cues.
Forked tracks: If the track splits, we can deduce two things: either we just saw a particle decay or we saw ionization of an atom. Many particles are unstable and will decay into more stable particles. It can also be a possibility that some particle has just knocked off an electron from an atom and that electron forms a track of its own whereas the particle gets deflected towards some other side.
Step 4: MATERIALS
- Clear plastic or glass tank (such as a fish tank)
- A metal sheet which can cover the glass chamber
- Felt
- Rubber
- Isopropyl alcohol (90% or more)
- Dry ice (frozen carbon dioxide)
- A hot water bag
- Vaseline
- Black chart paper
Step 5: PROCEDURE
- The back surface and one of the side surfaces were completely covered with black chart paper. The other side surface was covered in such a way that some space was left in the bottom for the torchlight to enter.
- For better visualization the metal plate was also painted black.
- A layer of tyre rubber was put around the edge of the open face of the glass tank to make the container air tight.
- The felt was cut so as to match the size of the bottom of the glass tank. It was then glued to inside of the tank (on the bottom surface).
- Once the felt was secured, it was soaked with isopropyl alcohol until it was saturated. Any excess alcohol was drained off. It may be possible that due to the weight of the soaked in alcohol, the felt may fall. To prevent this, the felt was secured with the help of wires. This was done before soaking the felt with alcohol.
- The glass tank was then placed over the metal plate so that the felt-covered bottom of the tank was on topside. Some vaseline was put around the joint to make the chamber air-tight.
- Now the tank along with the metal plate was placed over dry ice. It was made sure that the metal plate lies flat on the dry ice. The dry ice was kept in an ice-chest so that it was more stable.
- The metal plate soon became very cold because of the dry ice. Now the hot water bag was placed on top of the glass tank in order to create a steep temperature gradient.
- After 10 minutes lights were turned off and flashlight was shone into the bottom 2 inches of the tank.
Step 6: OBSERVATIONS
I made videos of the working cloud chamber. I've added the pictures showing the trails of various particles.
Step 7: PRECAUTIONS
- Handle dry ice with care. Always wear leather gloves while handling it to prevent ice burns.
- Dry ice releases CO2 gas. Hence do not keep it indoor.
- Handle isopropyl alcohol with care. Being extremely concentrated, its fumes can be highly irritable.
- Ensure that the chamber is airtight. Any leakage would cause the chamber to not work properly.
- Make sure that the water in the hot water bottle is hot enough to create a significant temperature gradient in the chamber.
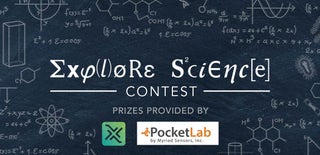
Participated in the
Explore Science Contest 2017
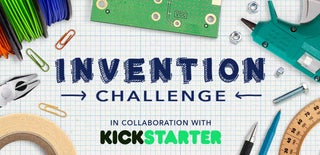
Participated in the
Invention Challenge 2017